Electrographic Features of Spontaneous Recurrent Seizures in a Mouse Model of Extended Hippocampal Kindling
- PMID: 34296153
- PMCID: PMC8152854
- DOI: 10.1093/texcom/tgab004
Electrographic Features of Spontaneous Recurrent Seizures in a Mouse Model of Extended Hippocampal Kindling
Abstract
Epilepsy is a chronic neurological disorder characterized by spontaneous recurrent seizures (SRS) and comorbidities. Kindling through repetitive brief stimulation of a limbic structure is a commonly used model of temporal lobe epilepsy. Particularly, extended kindling over a period up to a few months can induce SRS, which may simulate slowly evolving epileptogenesis of temporal lobe epilepsy. Currently, electroencephalographic (EEG) features of SRS in rodent models of extended kindling remain to be detailed. We explored this using a mouse model of extended hippocampal kindling. Intracranial EEG recordings were made from the kindled hippocampus and unstimulated hippocampal, neocortical, piriform, entorhinal, or thalamic area in individual mice. Spontaneous EEG discharges with concurrent low-voltage fast onsets were observed from the two corresponding areas in nearly all SRS detected, irrespective of associated motor seizures. Examined in brain slices, epileptiform discharges were induced by alkaline artificial cerebrospinal fluid in the hippocampal CA3, piriform and entorhinal cortical areas of extended kindled mice but not control mice. Together, these in vivo and in vitro observations suggest that the epileptic activity involving a macroscopic network may generate concurrent discharges in forebrain areas and initiate SRS in hippocampally kindled mice.
Keywords: brain slices; convulsion; epilepsy; ictal discharges; intracranial electroencephalograph.
© The Author(s) 2021. Published by Oxford University Press.
Figures
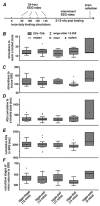
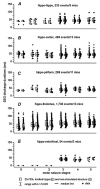
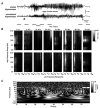
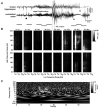
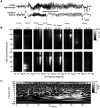
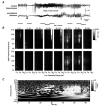
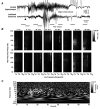
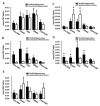
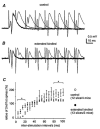
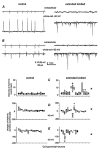
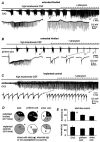
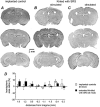
Similar articles
-
Convulsive behaviors of spontaneous recurrent seizures in a mouse model of extended hippocampal kindling.Front Behav Neurosci. 2022 Dec 23;16:1076718. doi: 10.3389/fnbeh.2022.1076718. eCollection 2022. Front Behav Neurosci. 2022. PMID: 36620863 Free PMC article.
-
Development of spontaneous recurrent seizures accompanied with increased rates of interictal spikes and decreased hippocampal delta and theta activities following extended kindling in mice.Exp Neurol. 2024 Sep;379:114860. doi: 10.1016/j.expneurol.2024.114860. Epub 2024 Jun 12. Exp Neurol. 2024. PMID: 38876195
-
Effects of Antiepileptic Drugs on Spontaneous Recurrent Seizures in a Novel Model of Extended Hippocampal Kindling in Mice.Front Pharmacol. 2018 May 18;9:451. doi: 10.3389/fphar.2018.00451. eCollection 2018. Front Pharmacol. 2018. PMID: 29867462 Free PMC article.
-
The role of the piriform cortex in kindling.Prog Neurobiol. 1996 Dec;50(5-6):427-81. doi: 10.1016/s0301-0082(96)00036-6. Prog Neurobiol. 1996. PMID: 9015822 Review.
-
Do interictal discharges promote or control seizures? Experimental evidence from an in vitro model of epileptiform discharge.Epilepsia. 2001;42 Suppl 3:2-4. doi: 10.1046/j.1528-1157.2001.042suppl.3002.x. Epilepsia. 2001. PMID: 11520313 Review.
Cited by
-
Convulsive behaviors of spontaneous recurrent seizures in a mouse model of extended hippocampal kindling.Front Behav Neurosci. 2022 Dec 23;16:1076718. doi: 10.3389/fnbeh.2022.1076718. eCollection 2022. Front Behav Neurosci. 2022. PMID: 36620863 Free PMC article.
-
Light, Kindling, Action! Brain-Wide Circuit Changes After Optogenetic Hippocampal Kindling Revealed by Functional Magnetic Resonance Imaging.Epilepsy Curr. 2022 Mar 26;22(3):190-191. doi: 10.1177/15357597221083405. eCollection 2022 Jun. Epilepsy Curr. 2022. PMID: 36474835 Free PMC article. No abstract available.
-
Anti-epileptic effect of 2-deoxy-D-glucose by activation of miR-194/KATP signaling pathway.Zhong Nan Da Xue Xue Bao Yi Xue Ban. 2022 Aug 28;47(8):1099-1107. doi: 10.11817/j.issn.1672-7347.2022.220111. Zhong Nan Da Xue Xue Bao Yi Xue Ban. 2022. PMID: 36097778 Free PMC article. Chinese, English.
-
Hyperexcitability: From Normal Fear to Pathological Anxiety and Trauma.Front Syst Neurosci. 2022 Aug 4;16:727054. doi: 10.3389/fnsys.2022.727054. eCollection 2022. Front Syst Neurosci. 2022. PMID: 35993088 Free PMC article. Review.
-
Clustering of Spontaneous Recurrent Seizures in a Mouse Model of Extended Hippocampal Kindling.Front Neurol. 2021 Nov 25;12:738986. doi: 10.3389/fneur.2021.738986. eCollection 2021. Front Neurol. 2021. PMID: 34899563 Free PMC article.
References
-
- Amaral DG, Witter MP. 1998. The three-dimensional organization of the hippocampal formation: a review of anatomical data. Neuroscience. 31:571–591. - PubMed
-
- Aronica E, Mühlebner A, Vliet EA, Gorter JA. 2017. In: Pitkänen A, Buckmaster PS, Galanopoulou AS, Moshé LS, editors. Models of seizures and epilepsy. 2nd ed. United Kingdom: Academic Press. pp. 139–160.
-
- Bertram EH. 2014. Extratemporal lobe circuits in temporal lobe epilepsy. Epilepsy Behav. 38:13–18. - PubMed
LinkOut - more resources
Full Text Sources
Miscellaneous