Insulin action at a molecular level - 100 years of progress
- PMID: 34274528
- PMCID: PMC8551477
- DOI: 10.1016/j.molmet.2021.101304
Insulin action at a molecular level - 100 years of progress
Abstract
The discovery of insulin 100 years ago and its application to the treatment of human disease in the years since have marked a major turning point in the history of medicine. The availability of purified insulin allowed for the establishment of its physiological role in the regulation of blood glucose and ketones, the determination of its amino acid sequence, and the solving of its structure. Over the last 50 years, the function of insulin has been applied into the discovery of the insulin receptor and its signaling cascade to reveal the role of impaired insulin signaling-or resistance-in the progression of type 2 diabetes. It has also become clear that insulin signaling can impact not only classical insulin-sensitive tissues, but all tissues of the body, and that in many of these tissues the insulin signaling cascade regulates unexpected physiological functions. Despite these remarkable advances, much remains to be learned about both insulin signaling and how to use this molecular knowledge to advance the treatment of type 2 diabetes and other insulin-resistant states.
Keywords: Insulin; Insulin receptor; Insulin resistance; Insulin signal transduction.
Copyright © 2021 The Authors. Published by Elsevier GmbH.. All rights reserved.
Conflict of interest statement
Conflict of interests M.F.W. is an advisory board member of Housey Pharma (https://www.housey.com/). C.R.K. is on the scientific advisory board or serves as a consultant for Kaleido Biosciences, CohBar, ERX Therapeutics, and Cellarity.
Figures
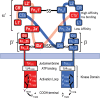
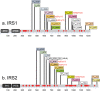
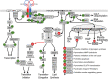
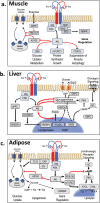
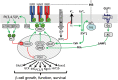
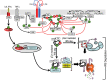
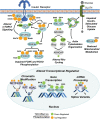
Similar articles
-
Role of reduced insulin-stimulated bone blood flow in the pathogenesis of metabolic insulin resistance and diabetic bone fragility.Med Hypotheses. 2016 Aug;93:81-6. doi: 10.1016/j.mehy.2016.05.008. Epub 2016 May 12. Med Hypotheses. 2016. PMID: 27372862
-
Insulin receptor signaling in normal and insulin-resistant states.Cold Spring Harb Perspect Biol. 2014 Jan 1;6(1):a009191. doi: 10.1101/cshperspect.a009191. Cold Spring Harb Perspect Biol. 2014. PMID: 24384568 Free PMC article. Review.
-
Reconstitution of insulin action in muscle, white adipose tissue, and brain of insulin receptor knock-out mice fails to rescue diabetes.J Biol Chem. 2011 Mar 18;286(11):9797-804. doi: 10.1074/jbc.M110.210807. Epub 2011 Jan 14. J Biol Chem. 2011. PMID: 21239487 Free PMC article.
-
Insulin action and resistance in obesity and type 2 diabetes.Nat Med. 2017 Jul 11;23(7):804-814. doi: 10.1038/nm.4350. Nat Med. 2017. PMID: 28697184 Free PMC article. Review.
-
Physiology. An integrative view of obesity.Science. 2007 Nov 9;318(5852):928-9. doi: 10.1126/science.1148032. Science. 2007. PMID: 17991852 No abstract available.
Cited by
-
Therapeutic opportunities in targeting the protective arm of the renin-angiotensin system to improve insulin sensitivity: a mechanistic review.Hypertens Res. 2024 Dec;47(12):3397-3408. doi: 10.1038/s41440-024-01909-y. Epub 2024 Oct 3. Hypertens Res. 2024. PMID: 39363004 Review.
-
Reduced insulin signaling in neurons induces sex-specific health benefits.Sci Adv. 2023 Feb 22;9(8):eade8137. doi: 10.1126/sciadv.ade8137. Epub 2023 Feb 22. Sci Adv. 2023. PMID: 36812323 Free PMC article.
-
The adipocyte supersystem of insulin and cAMP signaling.Trends Cell Biol. 2023 Apr;33(4):340-354. doi: 10.1016/j.tcb.2022.07.009. Epub 2022 Aug 18. Trends Cell Biol. 2023. PMID: 35989245 Free PMC article. Review.
-
The insulin centennial-100 years of milestones in biochemistry.J Lipid Res. 2021;62:100132. doi: 10.1016/j.jlr.2021.100132. Epub 2021 Oct 28. J Lipid Res. 2021. PMID: 34717951 Free PMC article. Review. No abstract available.
-
Association between type 2 diabetes mellitus and body composition based on MRI fat fraction mapping.Front Public Health. 2024 Jan 23;12:1332346. doi: 10.3389/fpubh.2024.1332346. eCollection 2024. Front Public Health. 2024. PMID: 38322122 Free PMC article.
References
-
- Kasuga M., Karlsson F.A., Kahn C.R. Insulin stimulates the phosphorylation of the 95,000-dalton subunit of its own receptor. Science. 1982;215(4529):185–187. - PubMed
-
- Bravo D.A., Gleason J.B., Sanchez R.I., Roth R.A., Fuller R.S. Accurate and efficient cleavage of the human insulin proreceptor by the human proprotein-processing protease furin. Characterization and kinetic parameters using the purified, secreted soluble protease expressed by a recombinant baculovirus. Journal of Biological Chemistry. 1994;269(41):25830–25837. - PubMed
-
- De Meyts P. Insulin/receptor binding: the last piece of the puzzle? What recent progress on the structure of the insulin/receptor complex tells us (or not) about negative cooperativity and activation. BioEssays. 2015;37(4):389–397. - PubMed
Publication types
MeSH terms
Substances
Grants and funding
LinkOut - more resources
Full Text Sources
Medical