SARS-CoV-2 infection induces the dedifferentiation of multiciliated cells and impairs mucociliary clearance
- PMID: 34272374
- PMCID: PMC8285531
- DOI: 10.1038/s41467-021-24521-x
SARS-CoV-2 infection induces the dedifferentiation of multiciliated cells and impairs mucociliary clearance
Abstract
Understanding how SARS-CoV-2 spreads within the respiratory tract is important to define the parameters controlling the severity of COVID-19. Here we examine the functional and structural consequences of SARS-CoV-2 infection in a reconstructed human bronchial epithelium model. SARS-CoV-2 replication causes a transient decrease in epithelial barrier function and disruption of tight junctions, though viral particle crossing remains limited. Rather, SARS-CoV-2 replication leads to a rapid loss of the ciliary layer, characterized at the ultrastructural level by axoneme loss and misorientation of remaining basal bodies. Downregulation of the master regulator of ciliogenesis Foxj1 occurs prior to extensive cilia loss, implicating this transcription factor in the dedifferentiation of ciliated cells. Motile cilia function is compromised by SARS-CoV-2 infection, as measured in a mucociliary clearance assay. Epithelial defense mechanisms, including basal cell mobilization and interferon-lambda induction, ramp up only after the initiation of cilia damage. Analysis of SARS-CoV-2 infection in Syrian hamsters further demonstrates the loss of motile cilia in vivo. This study identifies cilia damage as a pathogenic mechanism that could facilitate SARS-CoV-2 spread to the deeper lung parenchyma.
© 2021. The Author(s).
Conflict of interest statement
The authors declare no competing interests.
Figures
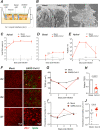
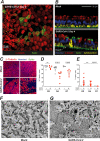
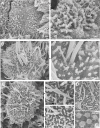
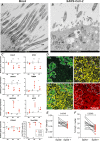
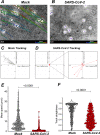
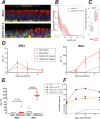
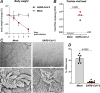
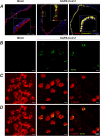
Similar articles
-
SARS-CoV-2 Infection Dysregulates Cilia and Basal Cell Homeostasis in the Respiratory Epithelium of Hamsters.Int J Mol Sci. 2022 May 4;23(9):5124. doi: 10.3390/ijms23095124. Int J Mol Sci. 2022. PMID: 35563514 Free PMC article.
-
Long-Term Modeling of SARS-CoV-2 Infection of In Vitro Cultured Polarized Human Airway Epithelium.mBio. 2020 Nov 6;11(6):e02852-20. doi: 10.1128/mBio.02852-20. mBio. 2020. PMID: 33158999 Free PMC article.
-
Live imaging of airway epithelium reveals that mucociliary clearance modulates SARS-CoV-2 spread.Nat Commun. 2024 Nov 2;15(1):9480. doi: 10.1038/s41467-024-53791-4. Nat Commun. 2024. PMID: 39488529 Free PMC article.
-
SARS-CoV replication and pathogenesis in an in vitro model of the human conducting airway epithelium.Virus Res. 2008 Apr;133(1):33-44. doi: 10.1016/j.virusres.2007.03.013. Epub 2007 Apr 23. Virus Res. 2008. PMID: 17451829 Free PMC article. Review.
-
Motile cilia and airway disease.Semin Cell Dev Biol. 2021 Feb;110:19-33. doi: 10.1016/j.semcdb.2020.11.007. Epub 2020 Dec 2. Semin Cell Dev Biol. 2021. PMID: 33279404 Review.
Cited by
-
SARS-CoV-2 infection of airway organoids reveals conserved use of Tetraspanin-8 by Ancestral, Delta, and Omicron variants.Stem Cell Reports. 2023 Mar 14;18(3):636-653. doi: 10.1016/j.stemcr.2023.01.011. Epub 2023 Feb 23. Stem Cell Reports. 2023. PMID: 36827975 Free PMC article.
-
Human Organotypic Airway and Lung Organoid Cells of Bronchiolar and Alveolar Differentiation Are Permissive to Infection by Influenza and SARS-CoV-2 Respiratory Virus.Front Cell Infect Microbiol. 2022 Mar 14;12:841447. doi: 10.3389/fcimb.2022.841447. eCollection 2022. Front Cell Infect Microbiol. 2022. PMID: 35360113 Free PMC article.
-
Mucociliary Clearance Augmenting Drugs Block SARS-Cov-2 Replication in Human Airway Epithelial Cells.bioRxiv [Preprint]. 2023 Feb 1:2023.01.30.526308. doi: 10.1101/2023.01.30.526308. bioRxiv. 2023. Update in: Am J Physiol Lung Cell Mol Physiol. 2023 Apr 1;324(4):L493-L506. doi: 10.1152/ajplung.00285.2022. PMID: 36778446 Free PMC article. Updated. Preprint.
-
NF-κB inhibitor alpha has a cross-variant role during SARS-CoV-2 infection in ACE2-overexpressing human airway organoids.bioRxiv [Preprint]. 2022 Aug 2:2022.08.02.502100. doi: 10.1101/2022.08.02.502100. bioRxiv. 2022. Update in: Sci Rep. 2024 Jul 4;14(1):15351. doi: 10.1038/s41598-024-66003-2. PMID: 35982664 Free PMC article. Updated. Preprint.
-
Cigarette smoke extract impairs gingival epithelial barrier function.Sci Rep. 2023 Jun 7;13(1):9228. doi: 10.1038/s41598-023-36366-z. Sci Rep. 2023. PMID: 37286570 Free PMC article.
References
Publication types
MeSH terms
Substances
LinkOut - more resources
Full Text Sources
Medical
Miscellaneous