Modulation of adenosine A2a receptor oligomerization by receptor activation and PIP2 interactions
- PMID: 34270937
- PMCID: PMC8581623
- DOI: 10.1016/j.str.2021.06.015
Modulation of adenosine A2a receptor oligomerization by receptor activation and PIP2 interactions
Abstract
GPCRs have been shown to form oligomers, which generate distinctive signaling outcomes. However, the structural nature of the oligomerization process remains uncertain. We have characterized oligomeric configurations of the adenosine A2a receptor (A2aR) by combining large-scale molecular dynamics simulations with Markov state models. These oligomeric structures may also serve as templates for studying oligomerization of other class A GPCRs. Our simulation data revealed that receptor activation results in enhanced oligomerization, more diverse oligomer populations, and a more connected oligomerization network. The active state conformation of the A2aR shifts protein-protein association interfaces to those involving intracellular loop ICL3 and transmembrane helix TM6. Binding of PIP2 to A2aR stabilizes protein-protein interactions via PIP2-mediated association interfaces. These results indicate that A2aR oligomerization is responsive to the local membrane lipid environment. This, in turn, suggests a modulatory effect on A2aR whereby a given oligomerization profile favors the dynamic formation of specific supramolecular signaling complexes.
Keywords: A2a receptor; GPCR; Markov state models; lipids; molecular dynamics; oligomerization.
Copyright © 2021 The Authors. Published by Elsevier Ltd.. All rights reserved.
Conflict of interest statement
Declaration of interests The authors declare no competing interests. M.S.P.S. is on the journal advisory board.
Figures
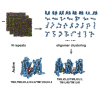
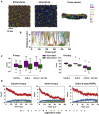
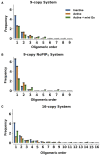
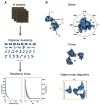

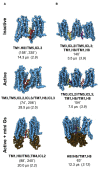
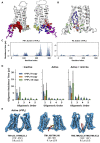
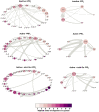
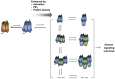
Similar articles
-
Homo-oligomerization of the human adenosine A2A receptor is driven by the intrinsically disordered C-terminus.Elife. 2021 Jul 16;10:e66662. doi: 10.7554/eLife.66662. Elife. 2021. PMID: 34269678 Free PMC article.
-
State-dependent Lipid Interactions with the A2a Receptor Revealed by MD Simulations Using In Vivo-Mimetic Membranes.Structure. 2019 Feb 5;27(2):392-403.e3. doi: 10.1016/j.str.2018.10.024. Epub 2018 Dec 20. Structure. 2019. PMID: 30581046 Free PMC article.
-
Molecular Biophysics of Class A G Protein Coupled Receptors-Lipids Interactome at a Glance-Highlights from the A2A Adenosine Receptor.Biomolecules. 2023 Jun 7;13(6):957. doi: 10.3390/biom13060957. Biomolecules. 2023. PMID: 37371538 Free PMC article. Review.
-
Dynamic behavior of the active and inactive states of the adenosine A(2A) receptor.J Phys Chem B. 2014 Mar 27;118(12):3355-65. doi: 10.1021/jp411618h. Epub 2014 Mar 17. J Phys Chem B. 2014. PMID: 24579769 Free PMC article.
-
The coming together of allosteric and phosphorylation mechanisms in the molecular integration of A2A heteroreceptor complexes in the dorsal and ventral striatal-pallidal GABA neurons.Pharmacol Rep. 2021 Aug;73(4):1096-1108. doi: 10.1007/s43440-021-00314-3. Epub 2021 Aug 24. Pharmacol Rep. 2021. PMID: 34426901 Free PMC article. Review.
Cited by
-
PI(4,5)P2: signaling the plasma membrane.Biochem J. 2022 Nov 11;479(21):2311-2325. doi: 10.1042/BCJ20220445. Biochem J. 2022. PMID: 36367756 Free PMC article.
-
Membrane Heteroreceptor Complexes as Second-Order Protein Modulators: A Novel Integrative Mechanism through Allosteric Receptor-Receptor Interactions.Membranes (Basel). 2024 Apr 25;14(5):96. doi: 10.3390/membranes14050096. Membranes (Basel). 2024. PMID: 38786931 Free PMC article.
-
Development of enhanced conformational sampling methods to probe the activation landscape of GPCRs.Adv Protein Chem Struct Biol. 2022;128:325-359. doi: 10.1016/bs.apcsb.2021.11.001. Epub 2021 Dec 20. Adv Protein Chem Struct Biol. 2022. PMID: 35034722 Free PMC article. Review.
-
Simulation-predicted and -explained inheritance model of pathogenicity confirmed by transgenic mice models.Comput Struct Biotechnol J. 2023 Nov 18;21:5698-5711. doi: 10.1016/j.csbj.2023.11.026. eCollection 2023. Comput Struct Biotechnol J. 2023. PMID: 38074473 Free PMC article.
-
Cardiolipin occupancy profiles of YidC paralogs reveal the significance of respective TM2 helix residues in determining paralog-specific phenotypes.Front Mol Biosci. 2023 Oct 6;10:1264454. doi: 10.3389/fmolb.2023.1264454. eCollection 2023. Front Mol Biosci. 2023. PMID: 37867558 Free PMC article.
References
-
- Abraham M.J., Murtola T., Schulz R., Páll S., Smith J.C., Hess B., Lindahl E. GROMACS: high performance molecular simulations through multi-level parallelism from laptops to supercomputers. SoftwareX. 2015;1–2:19–25.
-
- Calebiro D., Rieken F., Wagner J., Sungkaworn T., Zabel U., Borzi A., Cocucci E., Zurn A., Lohse M.J. Single-molecule analysis of fluorescently labeled G-protein-coupled receptors reveals complexes with distinct dynamics and organization. Proc. Natl. Acad. Sci. U S A. 2013;110:743–748. - PMC - PubMed
-
- Calebiro D., Sungkaworn T. Single-molecule imaging of GPCR interactions. Trends Pharmacol. Sci. 2018;39:109–122. - PubMed
Publication types
MeSH terms
Substances
Grants and funding
LinkOut - more resources
Full Text Sources