Meiotic recombination mirrors patterns of germline replication in mice and humans
- PMID: 34260899
- PMCID: PMC8591710
- DOI: 10.1016/j.cell.2021.06.025
Meiotic recombination mirrors patterns of germline replication in mice and humans
Abstract
Genetic recombination generates novel trait combinations, and understanding how recombination is distributed across the genome is key to modern genetics. The PRDM9 protein defines recombination hotspots; however, megabase-scale recombination patterning is independent of PRDM9. The single round of DNA replication, which precedes recombination in meiosis, may establish these patterns; therefore, we devised an approach to study meiotic replication that includes robust and sensitive mapping of replication origins. We find that meiotic DNA replication is distinct; reduced origin firing slows replication in meiosis, and a distinctive replication pattern in human males underlies the subtelomeric increase in recombination. We detected a robust correlation between replication and both contemporary and historical recombination and found that replication origin density coupled with chromosome size determines the recombination potential of individual chromosomes. Our findings and methods have implications for understanding the mechanisms underlying DNA replication, genetic recombination, and the landscape of mammalian germline variation.
Keywords: DNA replication; chromosome structure; crossover; genome evolution; genome stability; germline; in silico modeling; meiosis; recombination.
Published by Elsevier Inc.
Conflict of interest statement
Declaration of interests The authors declare no competing interests.
Figures
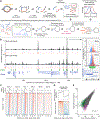
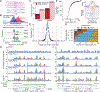
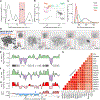
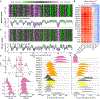
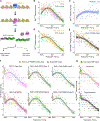
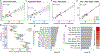
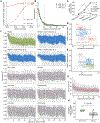
Comment in
-
First come, first served: Mammalian recombination is timed to replication.Cell. 2021 Aug 5;184(16):4112-4114. doi: 10.1016/j.cell.2021.07.014. Cell. 2021. PMID: 34358467
Similar articles
-
Analysis of Meiotic Double-Strand Break Initiation in Mammals.Methods Enzymol. 2018;601:391-418. doi: 10.1016/bs.mie.2017.11.037. Epub 2018 Feb 26. Methods Enzymol. 2018. PMID: 29523240 Free PMC article.
-
Genome-wide variability in recombination activity is associated with meiotic chromatin organization.Genome Res. 2021 Sep;31(9):1561-1572. doi: 10.1101/gr.275358.121. Epub 2021 Jul 23. Genome Res. 2021. PMID: 34301629 Free PMC article.
-
ZCWPW1 is recruited to recombination hotspots by PRDM9 and is essential for meiotic double strand break repair.Elife. 2020 Aug 3;9:e53392. doi: 10.7554/eLife.53392. Elife. 2020. PMID: 32744506 Free PMC article.
-
Meiotic recombination hotspots - a comparative view.Plant J. 2015 Jul;83(1):52-61. doi: 10.1111/tpj.12870. Epub 2015 May 20. Plant J. 2015. PMID: 25925869 Review.
-
Mammalian Meiotic Recombination: A Toolbox for Genome Evolution.Cytogenet Genome Res. 2016;150(1):1-16. doi: 10.1159/000452822. Epub 2016 Dec 8. Cytogenet Genome Res. 2016. PMID: 27926907 Review.
Cited by
-
Cell cycle gene alterations associate with a redistribution of mutation risk across chromosomal domains in human cancers.Nat Cancer. 2024 Feb;5(2):330-346. doi: 10.1038/s43018-023-00707-8. Epub 2024 Jan 10. Nat Cancer. 2024. PMID: 38200245
-
Crossover patterning in plants.Plant Reprod. 2023 Mar;36(1):55-72. doi: 10.1007/s00497-022-00445-4. Epub 2022 Jul 14. Plant Reprod. 2023. PMID: 35834006 Free PMC article. Review.
-
Characterization of Unidirectional Replication Forks in the Mouse Genome.Int J Mol Sci. 2023 Jun 1;24(11):9611. doi: 10.3390/ijms24119611. Int J Mol Sci. 2023. PMID: 37298562 Free PMC article.
-
Meiotic chromosome organization and its role in recombination and cancer.Curr Top Dev Biol. 2023;151:91-126. doi: 10.1016/bs.ctdb.2022.04.008. Epub 2022 Jun 20. Curr Top Dev Biol. 2023. PMID: 36681479 Free PMC article. Review.
-
Meiotic DNA breaks drive multifaceted mutagenesis in the human germ line.Science. 2023 Dec;382(6674):eadh2531. doi: 10.1126/science.adh2531. Epub 2023 Dec 1. Science. 2023. PMID: 38033082 Free PMC article.
References
-
- Baudat F, Manova K, Yuen JP, Jasin M, and Keeney S (2000). Chromosome synapsis defects and sexually dimorphic meiotic progression in mice lacking Spo11. Mol. Cell 6, 989–998. - PubMed
Publication types
MeSH terms
Grants and funding
LinkOut - more resources
Full Text Sources
Other Literature Sources
Molecular Biology Databases
Research Materials