Comparison of electrophysiological and motility assays to study anthelmintic effects in Caenorhabditis elegans
- PMID: 34252686
- PMCID: PMC8350797
- DOI: 10.1016/j.ijpddr.2021.05.005
Comparison of electrophysiological and motility assays to study anthelmintic effects in Caenorhabditis elegans
Abstract
Currently, only a few chemical drug classes are available to control the global burden of nematode infections in humans and animals. Most of these drugs exert their anthelmintic activity by interacting with proteins such as ion channels, and the nematode neuromuscular system remains a promising target for novel intervention strategies. Many commonly-used phenotypic readouts such as motility provide only indirect insight into neuromuscular function and the site(s) of action of chemical compounds. Electrophysiological recordings provide more specific information but are typically technically challenging and lack high throughput for drug discovery. Because drug discovery relies strongly on the evaluation and ranking of drug candidates, including closely related chemical derivatives, precise assays and assay combinations are needed for capturing and distinguishing subtle drug effects. Past studies show that nematode motility and pharyngeal pumping (feeding) are inhibited by most anthelmintic drugs. Here we compare two microfluidic devices ("chips") that record electrophysiological signals from the nematode pharynx (electropharyngeograms; EPGs) ─ the ScreenChip™ and the 8-channel EPG platform ─ to evaluate their respective utility for anthelmintic research. We additionally compared EPG data with whole-worm motility measurements obtained with the wMicroTracker instrument. As references, we used three macrocyclic lactones (ivermectin, moxidectin, and milbemycin oxime), and levamisole, which act on different ion channels. Drug potencies (IC50 and IC95 values) from concentration-response curves, and the time-course of drug effects, were compared across platforms and across drugs. Drug effects on pump timing and EPG waveforms were also investigated. These experiments confirmed drug-class specific effects of the tested anthelmintics and illustrated the relative strengths and limitations of the different assays for anthelmintic research.
Keywords: 8-channel chip; Anthelmintics; Caenorhabditis elegans; Electropharyngeogram (EPG); Levamisole; Macrocyclic lactones; Nematode pharynx; Pharyngeal pumping; ScreenChip; wMicroTracker.
Copyright © 2021 The Author(s). Published by Elsevier Ltd.. All rights reserved.
Conflict of interest statement
Iring Heisler is an employee of Elanco Animal Health and Daniel Kulke, and Steffen R. Hahnel were employees of Elanco Animal Health at the time the work was undertaken. Elanco Animal Health develops and sells veterinary pharmaceuticals including dewormers. Janis C. Weeks and William M. Roberts hold equity in InVivo Biosystems Inc., a company that develops and sells laboratory devices such as microfluidic EPG chip platforms and the wMicroTracker reported here. Except for the authors, Elanco Animal Health and InVivo Biosystems Inc. were not involved in the preparation of the manuscript. The decision to publish the manuscript was jointly taken.
Figures
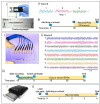
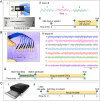
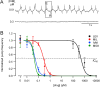
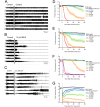
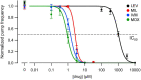
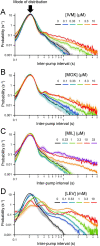
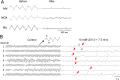
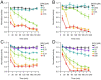
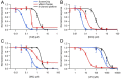
Similar articles
-
Anthelmintic drug actions in resistant and susceptible C. elegans revealed by electrophysiological recordings in a multichannel microfluidic device.Int J Parasitol Drugs Drug Resist. 2018 Dec;8(3):607-628. doi: 10.1016/j.ijpddr.2018.10.003. Epub 2018 Oct 30. Int J Parasitol Drugs Drug Resist. 2018. PMID: 30503202 Free PMC article.
-
Microfluidic platform for electrophysiological recordings from host-stage hookworm and Ascaris suum larvae: A new tool for anthelmintic research.Int J Parasitol Drugs Drug Resist. 2016 Dec;6(3):314-328. doi: 10.1016/j.ijpddr.2016.08.001. Epub 2016 Sep 15. Int J Parasitol Drugs Drug Resist. 2016. PMID: 27751868 Free PMC article.
-
Anthelmintic activity of KSI-4088 against Caenorhabditis elegans.Parasitol Res. 2010 Jun;107(1):27-30. doi: 10.1007/s00436-010-1828-8. Epub 2010 Mar 23. Parasitol Res. 2010. PMID: 20309581
-
Drug discovery technologies: Caenorhabditis elegans as a model for anthelmintic therapeutics.Med Res Rev. 2020 Sep;40(5):1715-1753. doi: 10.1002/med.21668. Epub 2020 Mar 13. Med Res Rev. 2020. PMID: 32166776 Review.
-
Pharmacology of anthelmintic resistance.Parasitology. 1996;113 Suppl:S201-16. doi: 10.1017/s0031182000077982. Parasitology. 1996. PMID: 9051936 Review.
Cited by
-
Anthelmintic Activity and Cytotoxic Effects of Compounds Isolated from the Fruits of Ozoroa insignis Del. (Anacardiaceae).Biomolecules. 2021 Dec 17;11(12):1893. doi: 10.3390/biom11121893. Biomolecules. 2021. PMID: 34944537 Free PMC article.
-
Biochemometry identifies ostruthin as pluripotent antimicrobial and anthelmintic agent from masterwort.iScience. 2023 Aug 3;26(9):107523. doi: 10.1016/j.isci.2023.107523. eCollection 2023 Sep 15. iScience. 2023. PMID: 37636068 Free PMC article.
-
A validated high-throughput method for assaying rat lungworm (Angiostrongylus cantonensis) motility when challenged with potentially anthelmintic natural products from Hawaiian fungi.Parasitology. 2022 Mar 3;149(6):1-28. doi: 10.1017/S0031182022000191. Online ahead of print. Parasitology. 2022. PMID: 35236524 Free PMC article.
-
Quantifying metabolic activity of Ascaris suum L3 using resazurin reduction.Parasit Vectors. 2023 Jul 19;16(1):243. doi: 10.1186/s13071-023-05871-5. Parasit Vectors. 2023. PMID: 37468906 Free PMC article.
References
-
- Ardelli B.F., Stitt L.E., Tompkins J.B., Prichard R.K. A comparison of the effects of ivermectin and moxidectin on the nematode Caenorhabditis elegans. Vet. Parasitol. 2009;165:96–108. - PubMed
-
- Avery L., Horvitz H.R. Effects of starvation and neuroactive drugs on feeding in Caenorhabditis elegans. J. Exp. Zool. 1990;253:263–270. - PubMed
-
- Blanchard A., Guegnard F., Charvet C., Crisford A., Courtot E., Sauvé C., Harmache A., Duguet T., O'Connor V., Castagnone-Sereno P., Reaves B., Wolstenholme A.J., Beech R.N., Holden-Dye L., Neveu C. Deciphering the molecular determinants of cholinergic anthelmintic sensitivity in nematodes: when novel functional validation approaches highlight major differences between the model Caenorhabditis elegans and parasitic species. PLoS Pathog. 2018;14 - PMC - PubMed
MeSH terms
Substances
LinkOut - more resources
Full Text Sources
Research Materials
Miscellaneous