Targeting Small GTPases and Their Prenylation in Diabetes Mellitus
- PMID: 34236862
- PMCID: PMC8389838
- DOI: 10.1021/acs.jmedchem.1c00410
Targeting Small GTPases and Their Prenylation in Diabetes Mellitus
Abstract
A fundamental role of pancreatic β-cells to maintain proper blood glucose level is controlled by the Ras superfamily of small GTPases that undergo post-translational modifications, including prenylation. This covalent attachment with either a farnesyl or a geranylgeranyl group controls their localization, activity, and protein-protein interactions. Small GTPases are critical in maintaining glucose homeostasis acting in the pancreas and metabolically active tissues such as skeletal muscles, liver, or adipocytes. Hyperglycemia-induced upregulation of small GTPases suggests that inhibition of these pathways deserves to be considered as a potential therapeutic approach in treating T2D. This Perspective presents how inhibition of various points in the mevalonate pathway might affect protein prenylation and functioning of diabetes-affected tissues and contribute to chronic inflammation involved in diabetes mellitus (T2D) development. We also demonstrate the currently available molecular tools to decipher the mechanisms linking the mevalonate pathway's enzymes and GTPases with diabetes.
Conflict of interest statement
The authors declare no competing financial interest.
Figures
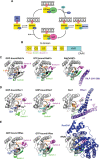
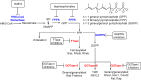
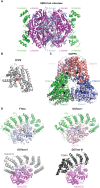
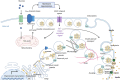
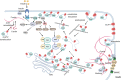
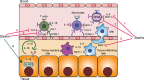
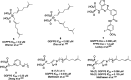
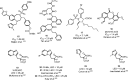
Similar articles
-
Simvastatin inhibits T-cell activation by selectively impairing the function of Ras superfamily GTPases.FASEB J. 2005 Apr;19(6):605-7. doi: 10.1096/fj.04-2702fje. Epub 2005 Jan 27. FASEB J. 2005. PMID: 15677697
-
PTP1b Inhibition, A Promising Approach for the Treatment of Diabetes Type II.Curr Top Med Chem. 2019;19(4):246-263. doi: 10.2174/1568026619666190201152153. Curr Top Med Chem. 2019. PMID: 30714526 Review.
-
Cardiac decompensation and promiscuous prenylation of small GTPases in cardiomyocytes in response to local mevalonate pathway disruption.J Pathol. 2022 Mar;256(3):249-252. doi: 10.1002/path.5837. Epub 2021 Dec 10. J Pathol. 2022. PMID: 34783037 Free PMC article.
-
The chaperone SmgGDS-607 has a dual role, both activating and inhibiting farnesylation of small GTPases.J Biol Chem. 2019 Aug 2;294(31):11793-11804. doi: 10.1074/jbc.RA119.007438. Epub 2019 Jun 13. J Biol Chem. 2019. PMID: 31197034 Free PMC article.
-
Inhibition of farnesyl pyrophosphate (FPP) and/or geranylgeranyl pyrophosphate (GGPP) biosynthesis and its implication in the treatment of cancers.Crit Rev Biochem Mol Biol. 2019 Feb;54(1):41-60. doi: 10.1080/10409238.2019.1568964. Epub 2019 Feb 18. Crit Rev Biochem Mol Biol. 2019. PMID: 30773935 Review.
Cited by
-
EMT activates exocytotic Rabs to coordinate invasion and immunosuppression in lung cancer.Proc Natl Acad Sci U S A. 2023 Jul 11;120(28):e2220276120. doi: 10.1073/pnas.2220276120. Epub 2023 Jul 5. Proc Natl Acad Sci U S A. 2023. PMID: 37406091 Free PMC article.
-
Brain Cholesterol Biosynthetic Pathway Is Altered in a Preclinical Model of Fragile X Syndrome.Int J Mol Sci. 2022 Mar 21;23(6):3408. doi: 10.3390/ijms23063408. Int J Mol Sci. 2022. PMID: 35328827 Free PMC article.
-
Statins Inhibit the Gliosis of MIO-M1, a Müller Glial Cell Line Induced by TRPV4 Activation.Int J Mol Sci. 2022 May 6;23(9):5190. doi: 10.3390/ijms23095190. Int J Mol Sci. 2022. PMID: 35563594 Free PMC article.
-
Focus on the Small GTPase Rab1: A Key Player in the Pathogenesis of Parkinson's Disease.Int J Mol Sci. 2021 Nov 8;22(21):12087. doi: 10.3390/ijms222112087. Int J Mol Sci. 2021. PMID: 34769517 Free PMC article. Review.
-
The Role of Rab GTPases in the development of genetic and malignant diseases.Mol Cell Biochem. 2024 Feb;479(2):255-281. doi: 10.1007/s11010-023-04727-x. Epub 2023 Apr 15. Mol Cell Biochem. 2024. PMID: 37060515 Review.
References
-
- Saeedi P.; Petersohn I.; Salpea P.; Malanda B.; Karuranga S.; Unwin N.; Colagiuri S.; Guariguata L.; Motala A. A.; Ogurtsova K.; Shaw J. E.; Bright D.; Williams R. Global and Regional Diabetes Prevalence Estimates for 2019 and Projections for 2030 and 2045: Results from the International Diabetes Federation Diabetes Atlas, 9th Edition. Diabetes Res. Clin. Pract. 2019, 157, 107843.10.1016/j.diabres.2019.107843. - DOI - PubMed
Publication types
MeSH terms
Substances
LinkOut - more resources
Full Text Sources
Medical