Synaptotagmin-7-mediated activation of spontaneous NMDAR currents is disrupted in bipolar disorder susceptibility variants
- PMID: 34228711
- PMCID: PMC8284830
- DOI: 10.1371/journal.pbio.3001323
Synaptotagmin-7-mediated activation of spontaneous NMDAR currents is disrupted in bipolar disorder susceptibility variants
Abstract
Synaptotagmin-7 (Syt7) plays direct or redundant Ca2+ sensor roles in multiple forms of vesicle exocytosis in synapses. Here, we show that Syt7 is a redundant Ca2+ sensor with Syt1/Doc2 to drive spontaneous glutamate release, which functions uniquely to activate the postsynaptic GluN2B-containing NMDARs that significantly contribute to mental illness. In mouse hippocampal neurons lacking Syt1/Doc2, Syt7 inactivation largely diminishes spontaneous release. Using 2 approaches, including measuring Ca2+ dose response and substituting extracellular Ca2+ with Sr2+, we detect that Syt7 directly triggers spontaneous release via its Ca2+ binding motif to activate GluN2B-NMDARs. Furthermore, modifying the localization of Syt7 in the active zone still allows Syt7 to drive spontaneous release, but the GluN2B-NMDAR activity is abolished. Finally, Syt7 SNPs identified in bipolar disorder patients destroy the function of Syt7 in spontaneous release in patient iPSC-derived and mouse hippocampal neurons. Therefore, Syt7 could contribute to neuropsychiatric disorders through driving spontaneous glutamate release.
Conflict of interest statement
The authors have declared that no competing interests exist.
Figures
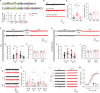
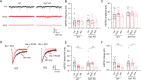
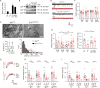
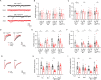
Similar articles
-
Synaptotagmin-7 deficiency induces mania-like behavioral abnormalities through attenuating GluN2B activity.Proc Natl Acad Sci U S A. 2020 Dec 8;117(49):31438-31447. doi: 10.1073/pnas.2016416117. Epub 2020 Nov 23. Proc Natl Acad Sci U S A. 2020. PMID: 33229564 Free PMC article.
-
Synaptotagmins 1 and 7 in vesicle release from rods of mouse retina.Exp Eye Res. 2022 Dec;225:109279. doi: 10.1016/j.exer.2022.109279. Epub 2022 Oct 22. Exp Eye Res. 2022. PMID: 36280223 Free PMC article.
-
Synaptotagmin-7 is a key factor for bipolar-like behavioral abnormalities in mice.Proc Natl Acad Sci U S A. 2020 Feb 25;117(8):4392-4399. doi: 10.1073/pnas.1918165117. Epub 2020 Feb 10. Proc Natl Acad Sci U S A. 2020. PMID: 32041882 Free PMC article.
-
Synaptotagmins 1 and 7 Play Complementary Roles in Somatodendritic Dopamine Release.J Neurosci. 2022 May 11;42(19):3919-3930. doi: 10.1523/JNEUROSCI.2416-21.2022. Epub 2022 Mar 31. J Neurosci. 2022. PMID: 35361702 Free PMC article.
-
Diverse roles of Synaptotagmin-7 in regulating vesicle fusion.Curr Opin Neurobiol. 2020 Aug;63:42-52. doi: 10.1016/j.conb.2020.02.006. Epub 2020 Apr 8. Curr Opin Neurobiol. 2020. PMID: 32278209 Free PMC article. Review.
Cited by
-
Pathological Interplay between Inflammation and Mitochondria Aggravates Glutamate Toxicity.Int J Mol Sci. 2024 Feb 14;25(4):2276. doi: 10.3390/ijms25042276. Int J Mol Sci. 2024. PMID: 38396952 Free PMC article. Review.
-
Phenotypes, mechanisms and therapeutics: insights from bipolar disorder GWAS findings.Mol Psychiatry. 2022 Jul;27(7):2927-2939. doi: 10.1038/s41380-022-01523-9. Epub 2022 Mar 29. Mol Psychiatry. 2022. PMID: 35351989 Review.
-
Vesicle trafficking and vesicle fusion: mechanisms, biological functions, and their implications for potential disease therapy.Mol Biomed. 2022 Sep 21;3(1):29. doi: 10.1186/s43556-022-00090-3. Mol Biomed. 2022. PMID: 36129576 Free PMC article. Review.
References
Publication types
MeSH terms
Substances
Grants and funding
LinkOut - more resources
Full Text Sources
Medical
Molecular Biology Databases
Miscellaneous