NOD-Like Receptors: Guards of Cellular Homeostasis Perturbation during Infection
- PMID: 34201509
- PMCID: PMC8268748
- DOI: 10.3390/ijms22136714
NOD-Like Receptors: Guards of Cellular Homeostasis Perturbation during Infection
Abstract
The innate immune system relies on families of pattern recognition receptors (PRRs) that detect distinct conserved molecular motifs from microbes to initiate antimicrobial responses. Activation of PRRs triggers a series of signaling cascades, leading to the release of pro-inflammatory cytokines, chemokines and antimicrobials, thereby contributing to the early host defense against microbes and regulating adaptive immunity. Additionally, PRRs can detect perturbation of cellular homeostasis caused by pathogens and fine-tune the immune responses. Among PRRs, nucleotide binding oligomerization domain (NOD)-like receptors (NLRs) have attracted particular interest in the context of cellular stress-induced inflammation during infection. Recently, mechanistic insights into the monitoring of cellular homeostasis perturbation by NLRs have been provided. We summarize the current knowledge about the disruption of cellular homeostasis by pathogens and focus on NLRs as innate immune sensors for its detection. We highlight the mechanisms employed by various pathogens to elicit cytoskeleton disruption, organelle stress as well as protein translation block, point out exemplary NLRs that guard cellular homeostasis during infection and introduce the concept of stress-associated molecular patterns (SAMPs). We postulate that integration of information about microbial patterns, danger signals, and SAMPs enables the innate immune system with adequate plasticity and precision in elaborating responses to microbes of variable virulence.
Keywords: NLRP1; NLRP3; NOD-like receptors; NOD1/2; cellular homeostasis; innate immunity; pathogens.
Conflict of interest statement
The authors declare no conflict of interest.
Figures
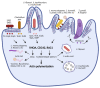

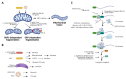
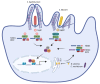
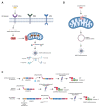
Similar articles
-
Activation and regulation mechanisms of NOD-like receptors based on structural biology.Front Immunol. 2022 Sep 15;13:953530. doi: 10.3389/fimmu.2022.953530. eCollection 2022. Front Immunol. 2022. PMID: 36189327 Free PMC article. Review.
-
NOD1 and NOD2 signalling links ER stress with inflammation.Nature. 2016 Apr 21;532(7599):394-7. doi: 10.1038/nature17631. Epub 2016 Mar 23. Nature. 2016. PMID: 27007849 Free PMC article.
-
An Overview of Pathogen Recognition Receptors for Innate Immunity in Dental Pulp.Mediators Inflamm. 2015;2015:794143. doi: 10.1155/2015/794143. Epub 2015 Oct 20. Mediators Inflamm. 2015. PMID: 26576076 Free PMC article. Review.
-
NOD1 and NOD2 Activation by Diverse Stimuli: a Possible Role for Sensing Pathogen-Induced Endoplasmic Reticulum Stress.Infect Immun. 2020 Jun 22;88(7):e00898-19. doi: 10.1128/IAI.00898-19. Print 2020 Jun 22. Infect Immun. 2020. PMID: 32229616 Free PMC article. Review.
-
Intracellular NOD-like receptors in innate immunity, infection and disease.Cell Microbiol. 2008 Jan;10(1):1-8. doi: 10.1111/j.1462-5822.2007.01059.x. Epub 2007 Oct 18. Cell Microbiol. 2008. PMID: 17944960 Review.
Cited by
-
Mettl3‑mediated m6A RNA methylation regulates osteolysis induced by titanium particles.Mol Med Rep. 2024 Mar;29(3):36. doi: 10.3892/mmr.2024.13160. Epub 2024 Jan 12. Mol Med Rep. 2024. PMID: 38214327 Free PMC article.
-
NOD1 and NOD2: Essential Monitoring Partners in the Innate Immune System.Curr Issues Mol Biol. 2024 Aug 28;46(9):9463-9479. doi: 10.3390/cimb46090561. Curr Issues Mol Biol. 2024. PMID: 39329913 Free PMC article. Review.
-
Human Endometrium Derived Mesenchymal Stem Cells with Aberrant NOD1 Expression Are Associated with Ectopic Endometrial Lesion Formation.Int J Stem Cells. 2024 Aug 30;17(3):309-318. doi: 10.15283/ijsc22200. Epub 2024 Mar 27. Int J Stem Cells. 2024. PMID: 38531608 Free PMC article.
-
NLRP3 inflammasome in digestive diseases: From mechanism to therapy.Front Immunol. 2022 Oct 26;13:978190. doi: 10.3389/fimmu.2022.978190. eCollection 2022. Front Immunol. 2022. PMID: 36389791 Free PMC article. Review.
-
The Roles of NOD-like Receptors in Innate Immunity in Otitis Media.Int J Mol Sci. 2022 Feb 21;23(4):2350. doi: 10.3390/ijms23042350. Int J Mol Sci. 2022. PMID: 35216465 Free PMC article. Review.
References
Publication types
MeSH terms
Substances
LinkOut - more resources
Full Text Sources