Use of Protamine in Nanopharmaceuticals-A Review
- PMID: 34200384
- PMCID: PMC8230241
- DOI: 10.3390/nano11061508
Use of Protamine in Nanopharmaceuticals-A Review
Abstract
Macromolecular biomolecules are currently dethroning classical small molecule therapeutics because of their improved targeting and delivery properties. Protamine-a small polycationic peptide-represents a promising candidate. In nature, it binds and protects DNA against degradation during spermatogenesis due to electrostatic interactions between the negatively charged DNA-phosphate backbone and the positively charged protamine. Researchers are mimicking this technique to develop innovative nanopharmaceutical drug delivery systems, incorporating protamine as a carrier for biologically active components such as DNA or RNA. The first part of this review highlights ongoing investigations in the field of protamine-associated nanotechnology, discussing the self-assembling manufacturing process and nanoparticle engineering. Immune-modulating properties of protamine are those that lead to the second key part, which is protamine in novel vaccine technologies. Protamine-based RNA delivery systems in vaccines (some belong to the new class of mRNA-vaccines) against infectious disease and their use in cancer treatment are reviewed, and we provide an update on the current state of latest developments with protamine as pharmaceutical excipient for vaccines.
Keywords: nanoparticles; novel vaccine technologies; protamine; proticles.
Conflict of interest statement
The authors declare no conflict of interest.
Figures
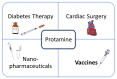
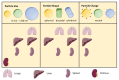
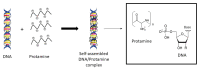
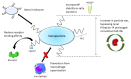
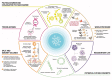
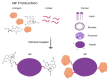
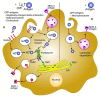
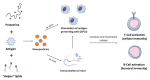
Similar articles
-
Protamine-oligonucleotide-nanoparticles: Recent advances in drug delivery and drug targeting.Eur J Pharm Sci. 2015 Jul 30;75:54-9. doi: 10.1016/j.ejps.2015.04.009. Epub 2015 Apr 17. Eur J Pharm Sci. 2015. PMID: 25896372 Review.
-
Depot formulation of vasoactive intestinal peptide by protamine-based biodegradable nanoparticles.J Control Release. 2008 Sep 10;130(2):192-8. doi: 10.1016/j.jconrel.2008.06.005. Epub 2008 Jun 14. J Control Release. 2008. PMID: 18601963
-
Manufacturing of a Secretoneurin Drug Delivery System with Self-Assembled Protamine Nanoparticles by Titration.PLoS One. 2016 Nov 9;11(11):e0164149. doi: 10.1371/journal.pone.0164149. eCollection 2016. PLoS One. 2016. PMID: 27828968 Free PMC article.
-
Cellular uptake and trafficking of peptide-based drug delivery systems for miRNA.Eur J Pharm Biopharm. 2023 Oct;191:189-204. doi: 10.1016/j.ejpb.2023.08.019. Epub 2023 Sep 4. Eur J Pharm Biopharm. 2023. PMID: 37666365
-
Protamine-Based Strategies for RNA Transfection.Pharmaceutics. 2021 Jun 14;13(6):877. doi: 10.3390/pharmaceutics13060877. Pharmaceutics. 2021. PMID: 34198550 Free PMC article. Review.
Cited by
-
PROTA: A Robust Tool for Protamine Prediction Using a Hybrid Approach of Machine Learning and Deep Learning.Int J Mol Sci. 2024 Sep 24;25(19):10267. doi: 10.3390/ijms251910267. Int J Mol Sci. 2024. PMID: 39408595 Free PMC article.
-
Nanomaterials in the Wound Healing Process: New Insights and Advancements.Pharmaceutics. 2024 Feb 21;16(3):300. doi: 10.3390/pharmaceutics16030300. Pharmaceutics. 2024. PMID: 38543194 Free PMC article. Review.
-
Engineering a Novel Modular Adenoviral mRNA Delivery Platform Based on Tag/Catcher Bioconjugation.Viruses. 2023 Nov 20;15(11):2277. doi: 10.3390/v15112277. Viruses. 2023. PMID: 38005953 Free PMC article.
-
mRNA vaccines and their delivery strategies: A journey from infectious diseases to cancer.Mol Ther. 2024 Jan 3;32(1):13-31. doi: 10.1016/j.ymthe.2023.10.024. Epub 2023 Nov 3. Mol Ther. 2024. PMID: 37919901 Review.
-
Protamine folds DNA into flowers and loop stacks.Biophys J. 2023 Nov 7;122(21):4288-4302. doi: 10.1016/j.bpj.2023.10.003. Epub 2023 Oct 6. Biophys J. 2023. PMID: 37803830
References
-
- Miescher F. Das Protamin, Eine Neue Organische Base Aus Den Samenfäden Des Rheinlachses. Ber. Dtsch. Chem. Ges. 1874;7:376–379. doi: 10.1002/cber.187400701119. - DOI
-
- Morkowin N. Ein Beitrag zur Kenntniss der Protamine. Hoppe-Seyler’s Z. Physiol. Chem. 1899;28:313–317. doi: 10.1515/bchm2.1899.28.3-4.313. - DOI
-
- Kossel A. Weitere Mittheilungen über die Protamine. Hoppe-Seyler’s Z. Physiol. Chem. 1899;26:588–592. doi: 10.1515/bchm2.1899.26.6.588. - DOI
Publication types
LinkOut - more resources
Full Text Sources