Molecular Details of the Frataxin-Scaffold Interaction during Mitochondrial Fe-S Cluster Assembly
- PMID: 34199378
- PMCID: PMC8199681
- DOI: 10.3390/ijms22116006
Molecular Details of the Frataxin-Scaffold Interaction during Mitochondrial Fe-S Cluster Assembly
Abstract
Iron-sulfur clusters are essential to almost every life form and utilized for their unique structural and redox-targeted activities within cells during many cellular pathways. Although there are three different Fe-S cluster assembly pathways in prokaryotes (the NIF, SUF and ISC pathways) and two in eukaryotes (CIA and ISC pathways), the iron-sulfur cluster (ISC) pathway serves as the central mechanism for providing 2Fe-2S clusters, directly and indirectly, throughout the entire cell in eukaryotes. Proteins central to the eukaryotic ISC cluster assembly complex include the cysteine desulfurase, a cysteine desulfurase accessory protein, the acyl carrier protein, the scaffold protein and frataxin (in humans, NFS1, ISD11, ACP, ISCU and FXN, respectively). Recent molecular details of this complex (labeled NIAUF from the first letter from each ISC protein outlined earlier), which exists as a dimeric pentamer, have provided real structural insight into how these partner proteins arrange themselves around the cysteine desulfurase, the core dimer of the (NIAUF)2 complex. In this review, we focus on both frataxin and the scaffold within the human, fly and yeast model systems to provide a better understanding of the biophysical characteristics of each protein alone and within the FXN/ISCU complex as it exists within the larger NIAUF construct. These details support a complex dynamic interaction between the FXN and ISCU proteins when both are part of the NIAUF complex and this provides additional insight into the coordinated mechanism of Fe-S cluster assembly.
Keywords: Fe-S cluster biosynthesis; ISC machinery; frataxin.
Conflict of interest statement
There are no conflicts of interest.
Figures
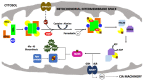
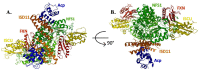
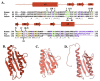
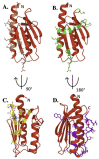
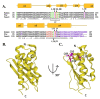
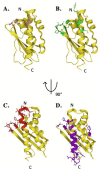
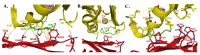
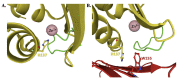
Similar articles
-
Structure of the human frataxin-bound iron-sulfur cluster assembly complex provides insight into its activation mechanism.Nat Commun. 2019 May 17;10(1):2210. doi: 10.1038/s41467-019-09989-y. Nat Commun. 2019. PMID: 31101807 Free PMC article.
-
Zinc(II) binding on human wild-type ISCU and Met140 variants modulates NFS1 desulfurase activity.Biochimie. 2018 Sep;152:211-218. doi: 10.1016/j.biochi.2018.07.012. Epub 2018 Jul 20. Biochimie. 2018. PMID: 30031876 Free PMC article.
-
Mammalian frataxin controls sulfur production and iron entry during de novo Fe4S4 cluster assembly.J Am Chem Soc. 2013 Jan 16;135(2):733-40. doi: 10.1021/ja308736e. Epub 2013 Jan 7. J Am Chem Soc. 2013. PMID: 23265191
-
Molecular characteristics of proteins within the mitochondrial Fe-S cluster assembly complex.Micron. 2022 Feb;153:103181. doi: 10.1016/j.micron.2021.103181. Epub 2021 Nov 12. Micron. 2022. PMID: 34823116 Review.
-
Mechanisms of Mitochondrial Iron-Sulfur Protein Biogenesis.Annu Rev Biochem. 2020 Jun 20;89:471-499. doi: 10.1146/annurev-biochem-013118-111540. Epub 2020 Jan 14. Annu Rev Biochem. 2020. PMID: 31935115 Review.
Cited by
-
Characteristics of the Isu1 C-terminus in relation to [2Fe-2S] cluster assembly and ISCU Myopathy.J Biol Inorg Chem. 2022 Dec;27(8):759-773. doi: 10.1007/s00775-022-01964-1. Epub 2022 Oct 30. J Biol Inorg Chem. 2022. PMID: 36309885
-
Searching for Frataxin Function: Exploring the Analogy with Nqo15, the Frataxin-like Protein of Respiratory Complex I from Thermus thermophilus.Int J Mol Sci. 2024 Feb 5;25(3):1912. doi: 10.3390/ijms25031912. Int J Mol Sci. 2024. PMID: 38339189 Free PMC article.
-
Recent Advances in the Elucidation of Frataxin Biochemical Function Open Novel Perspectives for the Treatment of Friedreich's Ataxia.Front Neurosci. 2022 Mar 2;16:838335. doi: 10.3389/fnins.2022.838335. eCollection 2022. Front Neurosci. 2022. PMID: 35310092 Free PMC article. Review.
-
Succinate Dehydrogenase, Succinate, and Superoxides: A Genetic, Epigenetic, Metabolic, Environmental Explosive Crossroad.Biomedicines. 2022 Jul 25;10(8):1788. doi: 10.3390/biomedicines10081788. Biomedicines. 2022. PMID: 35892689 Free PMC article. Review.
-
A Combined Spectroscopic and In Silico Approach to Evaluate the Interaction of Human Frataxin with Mitochondrial Superoxide Dismutase.Biomedicines. 2021 Nov 25;9(12):1763. doi: 10.3390/biomedicines9121763. Biomedicines. 2021. PMID: 34944579 Free PMC article.
References
-
- Srour B., Gervason S., Monfort B., D’Autreaux B. Mechanism of Iron-Slufur Cluster Assembly, In the Intimacy of Iron and SUlfur Encounter. Inorganics. 2020;8:55. doi: 10.3390/inorganics8100055. - DOI
Publication types
MeSH terms
Substances
Grants and funding
LinkOut - more resources
Full Text Sources
Medical
Molecular Biology Databases
Miscellaneous