Pathomechanisms and biomarkers in facioscapulohumeral muscular dystrophy: roles of DUX4 and PAX7
- PMID: 34151531
- PMCID: PMC8350899
- DOI: 10.15252/emmm.202013695
Pathomechanisms and biomarkers in facioscapulohumeral muscular dystrophy: roles of DUX4 and PAX7
Abstract
Facioscapulohumeral muscular dystrophy (FSHD) is characterised by progressive skeletal muscle weakness and wasting. FSHD is linked to epigenetic derepression of the subtelomeric D4Z4 macrosatellite at chromosome 4q35. Epigenetic derepression permits the distal-most D4Z4 unit to transcribe DUX4, with transcripts stabilised by splicing to a poly(A) signal on permissive 4qA haplotypes. The pioneer transcription factor DUX4 activates target genes that are proposed to drive FSHD pathology. While this toxic gain-of-function model is a satisfying "bottom-up" genotype-to-phenotype link, DUX4 is rarely detectable in muscle and DUX4 target gene expression is inconsistent in patients. A reliable biomarker for FSHD is suppression of a target gene score of PAX7, a master regulator of myogenesis. However, it is unclear how this "top-down" finding links to genomic changes that characterise FSHD and to DUX4. Here, we explore the roles and interactions of DUX4 and PAX7 in FSHD pathology and how the relationship between these two transcription factors deepens understanding via the immune system and muscle regeneration. Considering how FSHD pathomechanisms are represented by "DUX4opathy" models has implications for developing therapies and current clinical trials.
Keywords: DUX4; PAX7; biomarker; facioscapulohumeral muscular dystrophy (FSHD); pathology.
© 2021 The Authors. Published under the terms of the CC BY 4.0 license.
Conflict of interest statement
The authors declare that they have no conflict of interest.
Figures
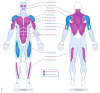
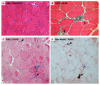

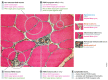
Similar articles
-
PAX7 target gene repression is a superior FSHD biomarker than DUX4 target gene activation, associating with pathological severity and identifying FSHD at the single-cell level.Hum Mol Genet. 2019 Jul 1;28(13):2224-2236. doi: 10.1093/hmg/ddz043. Hum Mol Genet. 2019. PMID: 31067297 Free PMC article.
-
PAX7 target genes are globally repressed in facioscapulohumeral muscular dystrophy skeletal muscle.Nat Commun. 2017 Dec 18;8(1):2152. doi: 10.1038/s41467-017-01200-4. Nat Commun. 2017. PMID: 29255294 Free PMC article.
-
PAX7 target gene repression associates with FSHD progression and pathology over 1 year.Hum Mol Genet. 2020 Aug 3;29(13):2124-2133. doi: 10.1093/hmg/ddaa079. Hum Mol Genet. 2020. PMID: 32347924
-
A complex interplay of genetic and epigenetic events leads to abnormal expression of the DUX4 gene in facioscapulohumeral muscular dystrophy.Neuromuscul Disord. 2016 Dec;26(12):844-852. doi: 10.1016/j.nmd.2016.09.015. Epub 2016 Sep 19. Neuromuscul Disord. 2016. PMID: 27816329 Review.
-
Facioscapulohumeral muscular dystrophy (FSHD): an enigma unravelled?Hum Genet. 2012 Mar;131(3):325-40. doi: 10.1007/s00439-011-1100-z. Epub 2011 Oct 9. Hum Genet. 2012. PMID: 21984394 Review.
Cited by
-
Molecular and Phenotypic Changes in FLExDUX4 Mice.J Pers Med. 2023 Jun 25;13(7):1040. doi: 10.3390/jpm13071040. J Pers Med. 2023. PMID: 37511653 Free PMC article.
-
FSHD muscle shows perturbation in fibroadipogenic progenitor cells, mitochondrial function and alternative splicing independently of inflammation.Hum Mol Genet. 2024 Jan 7;33(2):182-197. doi: 10.1093/hmg/ddad175. Hum Mol Genet. 2024. PMID: 37856562 Free PMC article.
-
DUX4 Role in Normal Physiology and in FSHD Muscular Dystrophy.Cells. 2021 Nov 26;10(12):3322. doi: 10.3390/cells10123322. Cells. 2021. PMID: 34943834 Free PMC article. Review.
-
The DUX4-HIF1α Axis in Murine and Human Muscle Cells: A Link More Complex Than Expected.Int J Mol Sci. 2024 Mar 15;25(6):3327. doi: 10.3390/ijms25063327. Int J Mol Sci. 2024. PMID: 38542301 Free PMC article.
-
Muscle pathology of antisynthetase syndrome according to antibody subtypes.Brain Pathol. 2023 Jul;33(4):e13155. doi: 10.1111/bpa.13155. Epub 2023 Mar 7. Brain Pathol. 2023. PMID: 36882048 Free PMC article. Review.
References
-
- Arahata K, Ishihara T, Fukunaga H, Orimo S, Lee JH, Goto K, Nonaka I (1995) Inflammatory response in facioscapulohumeral muscular dystrophy (FSHD): immunocytochemical and genetic analyses. Muscle Nerve 18(Supplement 2): S56–S66 - PubMed
-
- Bakay M, Wang Z, Melcon G, Schiltz L, Xuan J, Zhao P, Sartorelli V, Seo J, Pegoraro E, Angelini C et al (2006) Nuclear envelope dystrophies show a transcriptional fingerprint suggesting disruption of Rb‐MyoD pathways in muscle regeneration. Brain 129: 996–1013 - PubMed
Publication types
MeSH terms
Substances
Grants and funding
LinkOut - more resources
Full Text Sources
Other Literature Sources