Annexin A1 restores cerebrovascular integrity concomitant with reduced amyloid-β and tau pathology
- PMID: 34148071
- PMCID: PMC8262982
- DOI: 10.1093/brain/awab050
Annexin A1 restores cerebrovascular integrity concomitant with reduced amyloid-β and tau pathology
Abstract
Alzheimer's disease, characterized by brain deposits of amyloid-β plaques and neurofibrillary tangles, is also linked to neurovascular dysfunction and blood-brain barrier breakdown, affecting the passage of substances into and out of the brain. We hypothesized that treatment of neurovascular alterations could be beneficial in Alzheimer's disease. Annexin A1 (ANXA1) is a mediator of glucocorticoid anti-inflammatory action that can suppress microglial activation and reduce blood-brain barrier leakage. We have reported recently that treatment with recombinant human ANXA1 (hrANXA1) reduced amyloid-β levels by increased degradation in neuroblastoma cells and phagocytosis by microglia. Here, we show the beneficial effects of hrANXA1 in vivo by restoring efficient blood-brain barrier function and decreasing amyloid-β and tau pathology in 5xFAD mice and Tau-P301L mice. We demonstrate that young 5xFAD mice already suffer cerebrovascular damage, while acute pre-administration of hrANXA1 rescued the vascular defects. Interestingly, the ameliorated blood-brain barrier permeability in young 5xFAD mice by hrANXA1 correlated with reduced brain amyloid-β load, due to increased clearance and degradation of amyloid-β by insulin degrading enzyme (IDE). The systemic anti-inflammatory properties of hrANXA1 were also observed in 5xFAD mice, increasing IL-10 and reducing TNF-α expression. Additionally, the prolonged treatment with hrANXA1 reduced the memory deficits and increased synaptic density in young 5xFAD mice. Similarly, in Tau-P301L mice, acute hrANXA1 administration restored vascular architecture integrity, affecting the distribution of tight junctions, and reduced tau phosphorylation. The combined data support the hypothesis that blood-brain barrier breakdown early in Alzheimer's disease can be restored by hrANXA1 as a potential therapeutic approach.
Keywords: ANXA1; Aβ; BBB; IDE; tau.
© The Author(s) (2021). Published by Oxford University Press on behalf of the Guarantors of Brain.
Figures
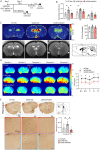
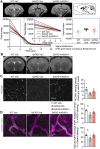
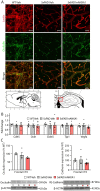
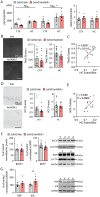
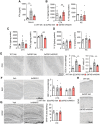
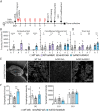
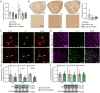
Similar articles
-
Annexin A1 restores Aβ1-42 -induced blood-brain barrier disruption through the inhibition of RhoA-ROCK signaling pathway.Aging Cell. 2017 Feb;16(1):149-161. doi: 10.1111/acel.12530. Epub 2016 Sep 16. Aging Cell. 2017. PMID: 27633771 Free PMC article.
-
The anti-inflammatory Annexin A1 induces the clearance and degradation of the amyloid-β peptide.J Neuroinflammation. 2016 Sep 2;13(1):234. doi: 10.1186/s12974-016-0692-6. J Neuroinflammation. 2016. PMID: 27590054 Free PMC article.
-
Crocus sativus Extract Tightens the Blood-Brain Barrier, Reduces Amyloid β Load and Related Toxicity in 5XFAD Mice.ACS Chem Neurosci. 2017 Aug 16;8(8):1756-1766. doi: 10.1021/acschemneuro.7b00101. Epub 2017 May 15. ACS Chem Neurosci. 2017. PMID: 28471166 Free PMC article.
-
Effects of CX3CR1 and Fractalkine Chemokines in Amyloid Beta Clearance and p-Tau Accumulation in Alzheimer's Disease (AD) Rodent Models: Is Fractalkine a Systemic Biomarker for AD?Curr Alzheimer Res. 2016;13(4):403-12. doi: 10.2174/1567205013666151116125714. Curr Alzheimer Res. 2016. PMID: 26567742 Review.
-
Dysfunction of the blood-brain barrier in Alzheimer's disease: Evidence from human studies.Neuropathol Appl Neurobiol. 2022 Apr;48(3):e12782. doi: 10.1111/nan.12782. Epub 2022 Feb 2. Neuropathol Appl Neurobiol. 2022. PMID: 34823269 Review.
Cited by
-
Advances in brain barriers and brain fluids research in 2021: great progress in a time of adversity.Fluids Barriers CNS. 2022 Jun 9;19(1):48. doi: 10.1186/s12987-022-00343-x. Fluids Barriers CNS. 2022. PMID: 35681151 Free PMC article.
-
Approaches for Increasing Cerebral Efflux of Amyloid-β in Experimental Systems.J Alzheimers Dis. 2024;100(2):379-411. doi: 10.3233/JAD-240212. J Alzheimers Dis. 2024. PMID: 38875041 Free PMC article. Review.
-
Preserved blood-brain barrier and neurovascular coupling in female 5xFAD model of Alzheimer's disease.Front Aging Neurosci. 2023 May 5;15:1089005. doi: 10.3389/fnagi.2023.1089005. eCollection 2023. Front Aging Neurosci. 2023. PMID: 37261266 Free PMC article.
-
Proteins and Transcriptional Dysregulation of the Brain Extracellular Matrix in Parkinson's Disease: A Systematic Review.Int J Mol Sci. 2023 Apr 18;24(8):7435. doi: 10.3390/ijms24087435. Int J Mol Sci. 2023. PMID: 37108598 Free PMC article. Review.
-
Surface protein profiling and subtyping of extracellular vesicles in body fluids reveals non-CSF biomarkers of Alzheimer's disease.J Extracell Vesicles. 2024 Apr;13(4):e12432. doi: 10.1002/jev2.12432. J Extracell Vesicles. 2024. PMID: 38602321 Free PMC article.
References
-
- Polanco JC, Li C, Bodea L-G, et al.Amyloid-β and tau complexity - towards improved biomarkers and targeted therapies. Nat Rev Neurol 2018;14:22-39. - PubMed
-
- Viggars AP, Wharton SB, Simpson JE, et al.Alterations in the blood brain barrier in ageing cerebral cortex in relationship to Alzheimer-type pathology: a study in the MRC-CFAS population neuropathology cohort. Neurosci Lett. 2011;505:25-30. - PubMed
Publication types
MeSH terms
Substances
Grants and funding
LinkOut - more resources
Full Text Sources
Medical
Molecular Biology Databases
Research Materials
Miscellaneous