Diabetic Endothelial Cells Differentiated From Patient iPSCs Show Dysregulated Glycine Homeostasis and Senescence Associated Phenotypes
- PMID: 34136485
- PMCID: PMC8201091
- DOI: 10.3389/fcell.2021.667252
Diabetic Endothelial Cells Differentiated From Patient iPSCs Show Dysregulated Glycine Homeostasis and Senescence Associated Phenotypes
Abstract
Induced pluripotent stem cells derived cells (iPSCs) not only can be used for personalized cell transfer therapy, but also can be used for modeling diseases for drug screening and discovery in vitro. Although prior studies have characterized the function of rodent iPSCs derived endothelial cells (ECs) in diabetes or metabolic syndrome, feature phenotypes are largely unknown in hiPSC-ECs from patients with diabetes. Here, we used hiPSC lines from patients with type 2 diabetes mellitus (T2DM) and differentiated them into ECs (dia-hiPSC-ECs). We found that dia-hiPSC-ECs had disrupted glycine homeostasis, increased senescence, and impaired mitochondrial function and angiogenic potential as compared with healthy hiPSC-ECs. These signature phenotypes will be helpful to establish dia-hiPSC-ECs as models of endothelial dysfunction for understanding molecular mechanisms of disease and for identifying and testing new targets for the treatment of endothelial dysfunction in diabetes.
Keywords: endothelial function; endothelium; glycine; mitochondrial function; senescence.
Copyright © 2021 Su, Kong, Loo, Gao, Kovalik, Su, Ma and Ye.
Conflict of interest statement
The authors declare that the research was conducted in the absence of any commercial or financial relationships that could be construed as a potential conflict of interest.
Figures
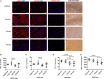
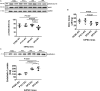
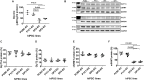
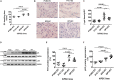
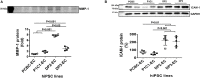
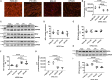
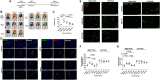
Similar articles
-
Thymosin beta-4 improves endothelial function and reparative potency of diabetic endothelial cells differentiated from patient induced pluripotent stem cells.Stem Cell Res Ther. 2022 Jan 10;13(1):13. doi: 10.1186/s13287-021-02687-x. Stem Cell Res Ther. 2022. PMID: 35012642 Free PMC article.
-
Supporting materials: Endothelial cells differentiated from patient dermal fibroblast-derived induced pluripotent stem cells resemble vascular malformations of Port Wine Birthmark.bioRxiv [Preprint]. 2023 Aug 24:2023.07.02.547408. doi: 10.1101/2023.07.02.547408. bioRxiv. 2023. Update in: Br J Dermatol. 2023 Nov 16;189(6):780-783. doi: 10.1093/bjd/ljad330 PMID: 37662218 Free PMC article. Updated. Preprint.
-
Human iPSCs-Derived Endothelial Cells with Mutation in HNF1A as a Model of Maturity-Onset Diabetes of the Young.Cells. 2019 Nov 14;8(11):1440. doi: 10.3390/cells8111440. Cells. 2019. PMID: 31739614 Free PMC article.
-
From bedside to the bench: patient-specific hiPSC-EC models uncover endothelial dysfunction in genetic cardiomyopathies.Front Physiol. 2023 Jul 19;14:1237101. doi: 10.3389/fphys.2023.1237101. eCollection 2023. Front Physiol. 2023. PMID: 37538375 Free PMC article. Review.
-
Recent Advances in Disease Modeling and Drug Discovery for Diabetes Mellitus Using Induced Pluripotent Stem Cells.Int J Mol Sci. 2016 Feb 19;17(2):256. doi: 10.3390/ijms17020256. Int J Mol Sci. 2016. PMID: 26907255 Free PMC article. Review.
Cited by
-
Vascular organoids: unveiling advantages, applications, challenges, and disease modelling strategies.Stem Cell Res Ther. 2023 Oct 10;14(1):292. doi: 10.1186/s13287-023-03521-2. Stem Cell Res Ther. 2023. PMID: 37817281 Free PMC article. Review.
-
Matrix metallopeptidase 9 contributes to the beginning of plaque and is a potential biomarker for the early identification of atherosclerosis in asymptomatic patients with diabetes.Front Endocrinol (Lausanne). 2024 Apr 10;15:1369369. doi: 10.3389/fendo.2024.1369369. eCollection 2024. Front Endocrinol (Lausanne). 2024. PMID: 38660518 Free PMC article.
-
Cellular interplay between cardiomyocytes and non-myocytes in diabetic cardiomyopathy.Cardiovasc Res. 2023 May 2;119(3):668-690. doi: 10.1093/cvr/cvac049. Cardiovasc Res. 2023. PMID: 35388880 Free PMC article. Review.
-
Thymosin beta-4 improves endothelial function and reparative potency of diabetic endothelial cells differentiated from patient induced pluripotent stem cells.Stem Cell Res Ther. 2022 Jan 10;13(1):13. doi: 10.1186/s13287-021-02687-x. Stem Cell Res Ther. 2022. PMID: 35012642 Free PMC article.
-
The Warburg effect alters amino acid homeostasis in human retinal endothelial cells: implication for proliferative diabetic retinopathy.Sci Rep. 2023 Sep 25;13(1):15973. doi: 10.1038/s41598-023-43022-z. Sci Rep. 2023. PMID: 37749155 Free PMC article.
References
-
- Christensen K., Roudnicky F., Burcin M., Patsch C. (2019). Monolayer generation of vascular endothelial cells from human pluripotent stem cells. Methods Mol. Biol. 1994 17–29. - PubMed
LinkOut - more resources
Full Text Sources
Other Literature Sources