Sorting Nexin 10 as a Key Regulator of Membrane Trafficking in Bone-Resorbing Osteoclasts: Lessons Learned From Osteopetrosis
- PMID: 34095139
- PMCID: PMC8173195
- DOI: 10.3389/fcell.2021.671210
Sorting Nexin 10 as a Key Regulator of Membrane Trafficking in Bone-Resorbing Osteoclasts: Lessons Learned From Osteopetrosis
Abstract
Bone homeostasis is a complex, multi-step process, which is based primarily on a tightly orchestrated interplay between bone formation and bone resorption that is executed by osteoblasts and osteoclasts (OCLs), respectively. The essential physiological balance between these cells is maintained and controlled at multiple levels, ranging from regulated gene expression to endocrine signals, yet the underlying cellular and molecular mechanisms are still poorly understood. One approach for deciphering the mechanisms that regulate bone homeostasis is the characterization of relevant pathological states in which this balance is disturbed. In this article we describe one such "error of nature," namely the development of acute recessive osteopetrosis (ARO) in humans that is caused by mutations in sorting nexin 10 (SNX10) that affect OCL functioning. We hypothesize here that, by virtue of its specific roles in vesicular trafficking, SNX10 serves as a key selective regulator of the composition of diverse membrane compartments in OCLs, thereby affecting critical processes in the sequence of events that link the plasma membrane with formation of the ruffled border and with extracellular acidification. As a result, SNX10 determines multiple features of these cells either directly or, as in regulation of cell-cell fusion, indirectly. This hypothesis is further supported by the similarities between the cellular defects observed in OCLs form various models of ARO, induced by mutations in SNX10 and in other genes, which suggest that mutations in the known ARO-associated genes act by disrupting the same plasma membrane-to-ruffled border axis, albeit to different degrees. In this article, we describe the population genetics and spread of the original arginine-to-glutamine mutation at position 51 (R51Q) in SNX10 in the Palestinian community. We further review recent studies, conducted in animal and cellular model systems, that highlight the essential roles of SNX10 in critical membrane functions in OCLs, and discuss possible future research directions that are needed for challenging or substantiating our hypothesis.
Keywords: ARO; SNX10; bone resorption; osteoclast; osteopetrosis; sorting nexin.
Copyright © 2021 Elson, Stein, Rabie, Barnea-Zohar, Winograd-Katz, Reuven, Shalev, Sekeres, Kanaan, Tuckermann and Geiger.
Conflict of interest statement
The authors declare that the research was conducted in the absence of any commercial or financial relationships that could be construed as a potential conflict of interest.
Figures
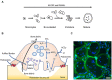
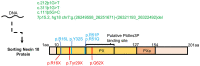
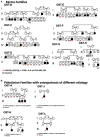
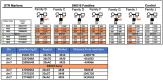
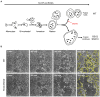
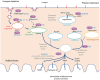
Similar articles
-
SNX10 regulates osteoclastogenic cell fusion and osteoclast size in mice.J Bone Miner Res. 2024 Sep 26;39(10):1503-1517. doi: 10.1093/jbmr/zjae125. J Bone Miner Res. 2024. PMID: 39095084
-
An SNX10-dependent mechanism downregulates fusion between mature osteoclasts.J Cell Sci. 2021 May 1;134(9):jcs254979. doi: 10.1242/jcs.254979. Epub 2021 May 11. J Cell Sci. 2021. PMID: 33975343 Free PMC article.
-
Massive osteopetrosis caused by non-functional osteoclasts in R51Q SNX10 mutant mice.Bone. 2020 Jul;136:115360. doi: 10.1016/j.bone.2020.115360. Epub 2020 Apr 8. Bone. 2020. PMID: 32278070
-
The molecular structure and function of sorting nexin 10 in skeletal disorders, cancers, and other pathological conditions.J Cell Physiol. 2021 Jun;236(6):4207-4215. doi: 10.1002/jcp.30173. Epub 2020 Nov 25. J Cell Physiol. 2021. PMID: 33241559 Review.
-
Osteopetrosis: genetics, treatment and new insights into osteoclast function.Nat Rev Endocrinol. 2013 Sep;9(9):522-36. doi: 10.1038/nrendo.2013.137. Epub 2013 Jul 23. Nat Rev Endocrinol. 2013. PMID: 23877423 Review.
Cited by
-
Using multi-scale genomics to associate poorly annotated genes with rare diseases.Genome Med. 2024 Jan 4;16(1):4. doi: 10.1186/s13073-023-01276-2. Genome Med. 2024. PMID: 38178268 Free PMC article.
-
Genome sequencing identifies a large non-coding region deletion of SNX10 causing autosomal recessive osteopetrosis.J Hum Genet. 2023 Apr;68(4):287-290. doi: 10.1038/s10038-022-01104-2. Epub 2022 Dec 16. J Hum Genet. 2023. PMID: 36526684 Free PMC article.
-
Molecular Heterogeneity of Osteopetrosis in India: Report of 17 Novel Variants.Indian J Hematol Blood Transfus. 2024 Jul;40(3):494-503. doi: 10.1007/s12288-023-01732-4. Epub 2024 Jan 12. Indian J Hematol Blood Transfus. 2024. PMID: 39011244
References
LinkOut - more resources
Full Text Sources
Research Materials
Miscellaneous