Sigma 1 Receptor Modulates Optic Nerve Head Astrocyte Reactivity
- PMID: 34086045
- PMCID: PMC8185400
- DOI: 10.1167/iovs.62.7.5
Sigma 1 Receptor Modulates Optic Nerve Head Astrocyte Reactivity
Abstract
Purpose: Stimulation of Sigma 1 Receptor (S1R) is neuroprotective in retina and optic nerve. S1R is expressed in both neurons and glia. The purpose of this work is to evaluate the ability of S1R to modulate reactivity responses of optic nerve head astrocytes (ONHAs) by investigating the extent to which S1R activation alters ONHA reactivity under conditions of ischemic cellular stress.
Methods: Wild type (WT) and S1R knockout (KO) ONHAs were derived and treated with vehicle or S1R agonist, (+)-pentazocine ((+)-PTZ). Cells were subjected to six hours of oxygen glucose deprivation (OGD) followed by 18 hours of re-oxygenation (OGD/R). Astrocyte reactivity responses were measured. Molecules that regulate ONHA reactivity, signal transducer and activator of transcription 3 (STAT3) and nuclear factor kappa B (NF-kB), were evaluated.
Results: Baseline glial fibrillary acidic protein (GFAP) levels were increased in nonstressed KO ONHAs compared with WT cultures. Baseline cellular migration was also increased in nonstressed KO ONHAs compared with WT. Treatment with (+)-PTZ increased cellular migration in nonstressed WT ONHAs but not in KO ONHAs. Exposure of both WT and KO ONHAs to ischemia (OGD/R), increased GFAP levels and cellular proliferation. However, (+)-PTZ treatment of OGD/R-exposed ONHAs enhanced GFAP levels, cellular proliferation, and cellular migration in WT but not KO cultures. The (+)-PTZ treatment of WT ONHAs also enhanced the OGD/R-induced increase in cellular pSTAT3 levels. However, treatment of WT ONHAs with (+)-PTZ abrogated the OGD/R-induced rise in NF-kB(p65) activation.
Conclusions: Under ischemic stress conditions, S1R activation enhanced ONHA reactivity characteristics. Future studies should address effects of these responses on RGC survival.
Conflict of interest statement
Disclosure:
Figures
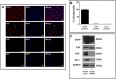
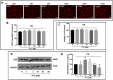
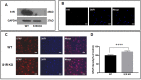
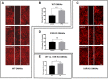
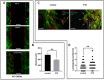
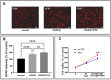
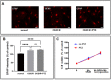
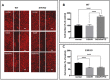
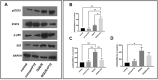
Similar articles
-
Sigma 1 Receptor Contributes to Astrocyte-Mediated Retinal Ganglion Cell Protection.Invest Ophthalmol Vis Sci. 2022 Feb 1;63(2):1. doi: 10.1167/iovs.63.2.1. Invest Ophthalmol Vis Sci. 2022. PMID: 35103752 Free PMC article.
-
Sigma 1 receptor regulates ERK activation and promotes survival of optic nerve head astrocytes.PLoS One. 2017 Sep 12;12(9):e0184421. doi: 10.1371/journal.pone.0184421. eCollection 2017. PLoS One. 2017. PMID: 28898265 Free PMC article.
-
Endothelin-1-induced proliferation is reduced and Ca²⁺ signaling is enhanced in endothelin B-deficient optic nerve head astrocytes.Invest Ophthalmol Vis Sci. 2011 Sep 29;52(10):7771-7. doi: 10.1167/iovs.11-7699. Invest Ophthalmol Vis Sci. 2011. PMID: 21873674
-
Peeking into Sigma-1 Receptor Functions Through the Retina.Adv Exp Med Biol. 2017;964:285-297. doi: 10.1007/978-3-319-50174-1_19. Adv Exp Med Biol. 2017. PMID: 28315278 Free PMC article. Review.
-
The Role of Sigma 1 Receptor as a Neuroprotective Target in Glaucoma.Adv Exp Med Biol. 2017;964:299-307. doi: 10.1007/978-3-319-50174-1_20. Adv Exp Med Biol. 2017. PMID: 28315279 Review.
Cited by
-
Sigma 1 Receptor Contributes to Astrocyte-Mediated Retinal Ganglion Cell Protection.Invest Ophthalmol Vis Sci. 2022 Feb 1;63(2):1. doi: 10.1167/iovs.63.2.1. Invest Ophthalmol Vis Sci. 2022. PMID: 35103752 Free PMC article.
-
SIGMAR1 Confers Innate Resilience against Neurodegeneration.Int J Mol Sci. 2023 Apr 24;24(9):7767. doi: 10.3390/ijms24097767. Int J Mol Sci. 2023. PMID: 37175473 Free PMC article. Review.
-
Therapeutic Properties of Ayahuasca Components in Ischemia/Reperfusion Injury of the Eye.Biomedicines. 2022 Apr 26;10(5):997. doi: 10.3390/biomedicines10050997. Biomedicines. 2022. PMID: 35625734 Free PMC article.
-
Mechanosensitive channel inhibition attenuates TGFβ2-induced actin cytoskeletal remodeling and reactivity in mouse optic nerve head astrocytes.Exp Eye Res. 2021 Nov;212:108791. doi: 10.1016/j.exer.2021.108791. Epub 2021 Oct 14. Exp Eye Res. 2021. PMID: 34656548 Free PMC article.
-
Long-Lasting Nociplastic Pain Modulation by Repeated Administration of Sigma-1 Receptor Antagonist BD1063 in Fibromyalgia-like Mouse Models.Int J Mol Sci. 2022 Oct 8;23(19):11933. doi: 10.3390/ijms231911933. Int J Mol Sci. 2022. PMID: 36233233 Free PMC article.
References
-
- Quigley HA, Addicks EM, Green WR, Maumenee AE.. Optic nerve damage in human glaucoma. II. The site of injury and susceptibility to damage. Arch Ophthalmol. 1981; 99: 635–649. - PubMed
-
- Quigley HA, Hohman RM, Addicks EM, Massof RW, Green WR.. Morphologic changes in the lamina cribrosa correlated with neural loss in open-angle glaucoma. Am J Ophthalmol. 1983; 95: 673–691. - PubMed
-
- Tham YC, Li X, Wong TY, Quigley HA, Aung T, Cheng CY.. Global prevalence of glaucoma and projections of glaucoma burden through 2040: a systematic review and meta-analysis. Ophthalmology. 2014; 121: 2081–2090. - PubMed
Publication types
MeSH terms
Substances
Grants and funding
LinkOut - more resources
Full Text Sources
Research Materials
Miscellaneous