DisA Limits RecG Activities at Stalled or Reversed Replication Forks
- PMID: 34073022
- PMCID: PMC8227628
- DOI: 10.3390/cells10061357
DisA Limits RecG Activities at Stalled or Reversed Replication Forks
Abstract
The DNA damage checkpoint protein DisA and the branch migration translocase RecG are implicated in the preservation of genome integrity in reviving haploid Bacillus subtilis spores. DisA synthesizes the essential cyclic 3', 5'-diadenosine monophosphate (c‑di-AMP) second messenger and such synthesis is suppressed upon replication perturbation. In vitro, c-di-AMP synthesis is suppressed when DisA binds DNA structures that mimic stalled or reversed forks (gapped forks or Holliday junctions [HJ]). RecG, which does not form a stable complex with DisA, unwinds branched intermediates, and in the presence of a limiting ATP concentration and HJ DNA, it blocks DisA-mediated c-di-AMP synthesis. DisA pre-bound to a stalled or reversed fork limits RecG-mediated ATP hydrolysis and DNA unwinding, but not if RecG is pre-bound to stalled or reversed forks. We propose that RecG-mediated fork remodeling is a genuine in vivo activity, and that DisA, as a molecular switch, limits RecG-mediated fork reversal and fork restoration. DisA and RecG might provide more time to process perturbed forks, avoiding genome breakage.
Keywords: DNA repair; DisA; RecG; c-di-AMP; stalled fork; template switching.
Conflict of interest statement
The authors declare no conflict of interest. The funders had no role in the design of the study; in the collection, analyses, or interpretation of data; in the writing of the manuscript, or in the decision to publish the results.
Figures
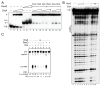
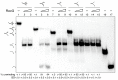
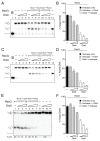
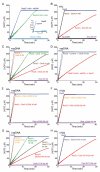
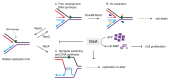
Similar articles
-
DisA Restrains the Processing and Cleavage of Reversed Replication Forks by the RuvAB-RecU Resolvasome.Int J Mol Sci. 2021 Oct 20;22(21):11323. doi: 10.3390/ijms222111323. Int J Mol Sci. 2021. PMID: 34768753 Free PMC article.
-
Activity and in vivo dynamics of Bacillus subtilis DisA are affected by RadA/Sms and by Holliday junction-processing proteins.DNA Repair (Amst). 2017 Jul;55:17-30. doi: 10.1016/j.dnarep.2017.05.002. Epub 2017 May 5. DNA Repair (Amst). 2017. PMID: 28511132
-
Bacillus subtilis DisA helps to circumvent replicative stress during spore revival.DNA Repair (Amst). 2017 Nov;59:57-68. doi: 10.1016/j.dnarep.2017.09.006. Epub 2017 Sep 22. DNA Repair (Amst). 2017. PMID: 28961460
-
Genome stability and the processing of damaged replication forks by RecG.Trends Genet. 2002 Aug;18(8):413-9. doi: 10.1016/s0168-9525(02)02720-8. Trends Genet. 2002. PMID: 12142010 Review.
-
DNA Helicase-SSB Interactions Critical to the Regression and Restart of Stalled DNA Replication forks in Escherichia coli.Genes (Basel). 2020 Apr 26;11(5):471. doi: 10.3390/genes11050471. Genes (Basel). 2020. PMID: 32357475 Free PMC article. Review.
Cited by
-
Processing of stalled replication forks in Bacillus subtilis.FEMS Microbiol Rev. 2024 Jan 12;48(1):fuad065. doi: 10.1093/femsre/fuad065. FEMS Microbiol Rev. 2024. PMID: 38052445 Free PMC article. Review.
-
Structure and kinase activity of bacterial cell cycle regulator CcrZ.PLoS Genet. 2022 May 16;18(5):e1010196. doi: 10.1371/journal.pgen.1010196. eCollection 2022 May. PLoS Genet. 2022. PMID: 35576203 Free PMC article.
-
DisA Restrains the Processing and Cleavage of Reversed Replication Forks by the RuvAB-RecU Resolvasome.Int J Mol Sci. 2021 Oct 20;22(21):11323. doi: 10.3390/ijms222111323. Int J Mol Sci. 2021. PMID: 34768753 Free PMC article.
-
Bacillus subtilis RecA, DisA, and RadA/Sms Interplay Prevents Replication Stress by Regulating Fork Remodeling.Front Microbiol. 2021 Nov 22;12:766897. doi: 10.3389/fmicb.2021.766897. eCollection 2021. Front Microbiol. 2021. PMID: 34880841 Free PMC article.
-
c-di-AMP signaling is required for bile salt resistance, osmotolerance, and long-term host colonization by Clostridioides difficile.Sci Signal. 2022 Sep 6;15(750):eabn8171. doi: 10.1126/scisignal.abn8171. Epub 2022 Sep 6. Sci Signal. 2022. PMID: 36067333 Free PMC article.
References
Publication types
MeSH terms
Substances
Grants and funding
LinkOut - more resources
Full Text Sources
Molecular Biology Databases