The Cellular and Chemical Biology of Endocytic Trafficking and Intracellular Delivery-The GL-Lect Hypothesis
- PMID: 34072622
- PMCID: PMC8198588
- DOI: 10.3390/molecules26113299
The Cellular and Chemical Biology of Endocytic Trafficking and Intracellular Delivery-The GL-Lect Hypothesis
Abstract
Lipid membranes are common to all forms of life. While being stable barriers that delimitate the cell as the fundamental organismal unit, biological membranes are highly dynamic by allowing for lateral diffusion, transbilayer passage via selective channels, and in eukaryotic cells for endocytic uptake through the formation of membrane bound vesicular or tubular carriers. Two of the most abundant fundamental fabrics of membranes-lipids and complex sugars-are produced through elaborate chains of biosynthetic enzymes, which makes it difficult to study them by conventional reverse genetics. This review illustrates how organic synthesis provides access to uncharted areas of membrane glycobiology research and its application to biomedicine. For this Special Issue on Chemical Biology Research in France, focus will be placed on synthetic approaches (i) to study endocytic functions of glycosylated proteins and lipids according to the GlycoLipid-Lectin (GL-Lect) hypothesis, notably that of Shiga toxin; (ii) to mechanistically dissect its endocytosis and intracellular trafficking with small molecule; and (iii) to devise intracellular delivery strategies for immunotherapy and tumor targeting. It will be pointed out how the chemical biologist's view on lipids, sugars, and proteins synergizes with biophysics and modeling to "look" into the membrane for atomistic scale insights on molecular rearrangements that drive the biogenesis of endocytic carriers in processes of clathrin-independent endocytosis.
Keywords: Casimir force; endocytosis; galectin; glycosphingolipid; glycosylation; immunotherapy; raft; retrograde trafficking; small molecule; tumor targeting.
Conflict of interest statement
The author declares no conflict of interest.
Figures
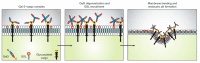
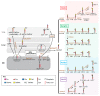
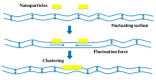
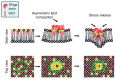
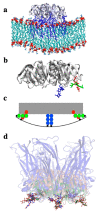
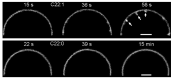
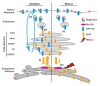
Similar articles
-
Shiga Toxin-A Model for Glycolipid-Dependent and Lectin-Driven Endocytosis.Toxins (Basel). 2017 Oct 25;9(11):340. doi: 10.3390/toxins9110340. Toxins (Basel). 2017. PMID: 29068384 Free PMC article. Review.
-
Glycosylation and raft endocytosis in cancer.Cancer Metastasis Rev. 2020 Jun;39(2):375-396. doi: 10.1007/s10555-020-09880-z. Cancer Metastasis Rev. 2020. PMID: 32388640 Free PMC article. Review.
-
Endocytic Roles of Glycans on Proteins and Lipids.Cold Spring Harb Perspect Biol. 2024 Jan 2;16(1):a041398. doi: 10.1101/cshperspect.a041398. Cold Spring Harb Perspect Biol. 2024. PMID: 37735065 Review.
-
Transcytosis of Galectin-3 in Mouse Intestine.Methods Mol Biol. 2022;2442:367-390. doi: 10.1007/978-1-0716-2055-7_20. Methods Mol Biol. 2022. PMID: 35320536
-
Exploration into Galectin-3 Driven Endocytosis and Lattices.Biomolecules. 2024 Sep 18;14(9):1169. doi: 10.3390/biom14091169. Biomolecules. 2024. PMID: 39334935 Free PMC article.
Cited by
-
Persistent Properties of a Subpopulation of Cancer Cells Overexpressing the Hedgehog Receptor Patched.Pharmaceutics. 2022 May 5;14(5):988. doi: 10.3390/pharmaceutics14050988. Pharmaceutics. 2022. PMID: 35631574 Free PMC article.
-
Cholera Toxin as a Probe for Membrane Biology.Toxins (Basel). 2021 Aug 3;13(8):543. doi: 10.3390/toxins13080543. Toxins (Basel). 2021. PMID: 34437414 Free PMC article. Review.
-
Oligosaccharide Presentation Modulates the Molecular Recognition of Glycolipids by Galectins on Membrane Surfaces.Pharmaceuticals (Basel). 2022 Jan 26;15(2):145. doi: 10.3390/ph15020145. Pharmaceuticals (Basel). 2022. PMID: 35215258 Free PMC article.
-
Generation of nanoscopic membrane curvature for membrane trafficking.Nat Rev Mol Cell Biol. 2023 Jan;24(1):63-78. doi: 10.1038/s41580-022-00511-9. Epub 2022 Aug 2. Nat Rev Mol Cell Biol. 2023. PMID: 35918535 Review.
-
Reduced endosomal microautophagy activity in aging associates with enhanced exocyst-mediated protein secretion.Aging Cell. 2022 Oct;21(10):e13713. doi: 10.1111/acel.13713. Epub 2022 Sep 18. Aging Cell. 2022. PMID: 36116133 Free PMC article.
References
Publication types
MeSH terms
Substances
LinkOut - more resources
Full Text Sources