Targeting CDK9 for Anti-Cancer Therapeutics
- PMID: 34062779
- PMCID: PMC8124690
- DOI: 10.3390/cancers13092181
Targeting CDK9 for Anti-Cancer Therapeutics
Abstract
Cyclin Dependent Kinase 9 (CDK9) is one of the most important transcription regulatory members of the CDK family. In conjunction with its main cyclin partner-Cyclin T1, it forms the Positive Transcription Elongation Factor b (P-TEFb) whose primary function in eukaryotic cells is to mediate the positive transcription elongation of nascent mRNA strands, by phosphorylating the S2 residues of the YSPTSPS tandem repeats at the C-terminus domain (CTD) of RNA Polymerase II (RNAP II). To aid in this process, P-TEFb also simultaneously phosphorylates and inactivates a number of negative transcription regulators like 5,6-dichloro-1-β-D-ribofuranosylbenzimidazole (DRB) Sensitivity-Inducing Factor (DSIF) and Negative Elongation Factor (NELF). Significantly enhanced activity of CDK9 is observed in multiple cancer types, which is universally associated with significantly shortened Overall Survival (OS) of the patients. In these cancer types, CDK9 regulates a plethora of cellular functions including proliferation, survival, cell cycle regulation, DNA damage repair and metastasis. Due to the extremely critical role of CDK9 in cancer cells, inhibiting its functions has been the subject of intense research, resulting the development of multiple, increasingly specific small-molecule inhibitors, some of which are presently in clinical trials. The search for newer generation CDK9 inhibitors with higher specificity and lower potential toxicities and suitable combination therapies continues. In fact, the Phase I clinical trials of the latest, highly specific CDK9 inhibitor BAY1251152, against different solid tumors have shown good anti-tumor and on-target activities and pharmacokinetics, combined with manageable safety profile while the phase I and II clinical trials of another inhibitor AT-7519 have been undertaken or are undergoing. To enhance the effectiveness and target diversity and reduce potential drug-resistance, the future of CDK9 inhibition would likely involve combining CDK9 inhibitors with inhibitors like those against BRD4, SEC, MYC, MCL-1 and HSP90.
Keywords: Apoptosis; BRD4; CDK9; Cyclin T1; MYC; RNAP II; Transcription.
Conflict of interest statement
The authors declare no conflict of interest.
Figures
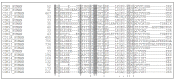
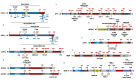
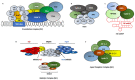
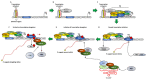
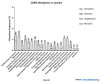
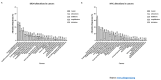
Similar articles
-
CDK9: a signaling hub for transcriptional control.Transcription. 2019 Apr;10(2):57-75. doi: 10.1080/21541264.2018.1523668. Epub 2018 Oct 11. Transcription. 2019. PMID: 30227759 Free PMC article. Review.
-
Positive transcription elongation factor b (P-TEFb) is a therapeutic target in human multiple myeloma.Oncotarget. 2017 Aug 1;8(35):59476-59491. doi: 10.18632/oncotarget.19761. eCollection 2017 Aug 29. Oncotarget. 2017. PMID: 28938651 Free PMC article.
-
Bromodomain protein Brd4 regulates human immunodeficiency virus transcription through phosphorylation of CDK9 at threonine 29.J Virol. 2009 Jan;83(2):1036-44. doi: 10.1128/JVI.01316-08. Epub 2008 Oct 29. J Virol. 2009. PMID: 18971272 Free PMC article.
-
The emerging picture of CDK9/P-TEFb: more than 20 years of advances since PITALRE.Mol Biosyst. 2017 Jan 31;13(2):246-276. doi: 10.1039/c6mb00387g. Mol Biosyst. 2017. PMID: 27833949 Review.
-
Tat modifies the activity of CDK9 to phosphorylate serine 5 of the RNA polymerase II carboxyl-terminal domain during human immunodeficiency virus type 1 transcription.Mol Cell Biol. 2000 Jul;20(14):5077-86. doi: 10.1128/MCB.20.14.5077-5086.2000. Mol Cell Biol. 2000. PMID: 10866664 Free PMC article.
Cited by
-
Management of Acute Myeloid Leukemia: Current Treatment Options and Future Perspectives.Cancers (Basel). 2021 Nov 16;13(22):5722. doi: 10.3390/cancers13225722. Cancers (Basel). 2021. PMID: 34830877 Free PMC article. Review.
-
CDK9 inhibitors in multiple myeloma: a review of progress and perspectives.Med Oncol. 2022 Jan 29;39(4):39. doi: 10.1007/s12032-021-01636-1. Med Oncol. 2022. PMID: 35092513 Free PMC article. Review.
-
DNMT1-mediated epigenetic suppression of FBXO32 expression promoting cyclin dependent kinase 9 (CDK9) survival and esophageal cancer cell growth.Cell Cycle. 2024 Feb;23(3):262-278. doi: 10.1080/15384101.2024.2309022. Epub 2024 Apr 10. Cell Cycle. 2024. PMID: 38597826
-
The BRD4 Inhibitor dBET57 Exerts Anticancer Effects by Targeting Superenhancer-Related Genes in Neuroblastoma.J Immunol Res. 2022 Nov 16;2022:7945884. doi: 10.1155/2022/7945884. eCollection 2022. J Immunol Res. 2022. PMID: 36438198 Free PMC article.
-
CDK9 binds and activates SGK3 to promote cardiac repair after injury via the GSK-3β/β-catenin pathway.Front Cardiovasc Med. 2022 Aug 23;9:970745. doi: 10.3389/fcvm.2022.970745. eCollection 2022. Front Cardiovasc Med. 2022. PMID: 36082129 Free PMC article.
References
-
- Cassandri M., Fioravanti R., Pomella S., Valente S., Rotili D., Del Baldo G., De Angelis B., Rota R., Mai A. CDK9 as a Valuable Target in Cancer: From Natural Compounds Inhibitors to Current Treatment in Pediatric Soft Tissue Sarcomas. Front. Pharmacol. 2020;11:1230. doi: 10.3389/fphar.2020.01230. - DOI - PMC - PubMed
Publication types
Grants and funding
LinkOut - more resources
Full Text Sources
Other Literature Sources
Miscellaneous