Lipolysis drives expression of the constitutively active receptor GPR3 to induce adipose thermogenesis
- PMID: 34048700
- PMCID: PMC8238500
- DOI: 10.1016/j.cell.2021.04.037
Lipolysis drives expression of the constitutively active receptor GPR3 to induce adipose thermogenesis
Abstract
Thermogenic adipocytes possess a therapeutically appealing, energy-expending capacity, which is canonically cold-induced by ligand-dependent activation of β-adrenergic G protein-coupled receptors (GPCRs). Here, we uncover an alternate paradigm of GPCR-mediated adipose thermogenesis through the constitutively active receptor, GPR3. We show that the N terminus of GPR3 confers intrinsic signaling activity, resulting in continuous Gs-coupling and cAMP production without an exogenous ligand. Thus, transcriptional induction of Gpr3 represents the regulatory parallel to ligand-binding of conventional GPCRs. Consequently, increasing Gpr3 expression in thermogenic adipocytes is alone sufficient to drive energy expenditure and counteract metabolic disease in mice. Gpr3 transcription is cold-stimulated by a lipolytic signal, and dietary fat potentiates GPR3-dependent thermogenesis to amplify the response to caloric excess. Moreover, we find GPR3 to be an essential, adrenergic-independent regulator of human brown adipocytes. Taken together, our findings reveal a noncanonical mechanism of GPCR control and thermogenic activation through the lipolysis-induced expression of constitutively active GPR3.
Keywords: G protein-coupled receptor; GPCR; GPR3; adrenergic receptor; brown adipose tissue; constitutively active; energy expenditure; lipolysis; thermogenesis; transcription.
Copyright © 2021 The Author(s). Published by Elsevier Inc. All rights reserved.
Conflict of interest statement
Declaration of interests O.S.J., Jakob Bondo Hansen, D.P.C., T.W.S., and Z.G.-H. work or have worked, in some capacity, for Embark Biotech ApS, a company developing therapeutics for the treatment of diabetes and obesity. All other authors declare no competing interests associated with this manuscript.
Figures
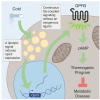
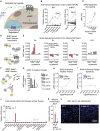
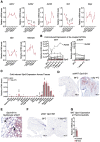
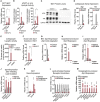
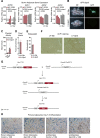
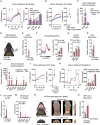
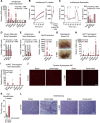
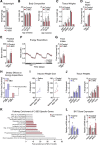
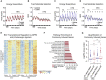
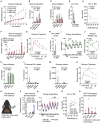
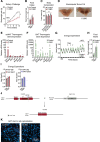
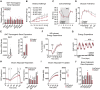
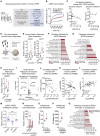
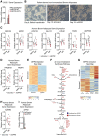
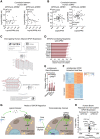
Comment in
-
GPR3 sets brown fat on fire.Cell Metab. 2021 Jul 6;33(7):1271-1273. doi: 10.1016/j.cmet.2021.06.003. Cell Metab. 2021. PMID: 34233169
-
Detouring adrenergic stimulation to induce adipose thermogenesis.Nat Rev Endocrinol. 2021 Oct;17(10):579-580. doi: 10.1038/s41574-021-00546-6. Nat Rev Endocrinol. 2021. PMID: 34326524 Free PMC article.
Similar articles
-
GPR3 sets brown fat on fire.Cell Metab. 2021 Jul 6;33(7):1271-1273. doi: 10.1016/j.cmet.2021.06.003. Cell Metab. 2021. PMID: 34233169
-
Mice lacking GPR3 receptors display late-onset obese phenotype due to impaired thermogenic function in brown adipose tissue.Sci Rep. 2015 Oct 12;5:14953. doi: 10.1038/srep14953. Sci Rep. 2015. PMID: 26455425 Free PMC article.
-
STAT5 is required for lipid breakdown and beta-adrenergic responsiveness of brown adipose tissue.Mol Metab. 2020 Oct;40:101026. doi: 10.1016/j.molmet.2020.101026. Epub 2020 May 28. Mol Metab. 2020. PMID: 32473405 Free PMC article.
-
Learning new tricks from old dogs: beta-adrenergic receptors teach new lessons on firing up adipose tissue metabolism.Mol Endocrinol. 2004 Sep;18(9):2123-31. doi: 10.1210/me.2004-0193. Epub 2004 Jul 8. Mol Endocrinol. 2004. PMID: 15243132 Review.
-
A compendium of G-protein-coupled receptors and cyclic nucleotide regulation of adipose tissue metabolism and energy expenditure.Clin Sci (Lond). 2020 Mar 13;134(5):473-512. doi: 10.1042/CS20190579. Clin Sci (Lond). 2020. PMID: 32149342 Free PMC article. Review.
Cited by
-
Adipose Tissue Remodeling in Pathophysiology.Annu Rev Pathol. 2023 Jan 24;18:71-93. doi: 10.1146/annurev-pathol-042220-023633. Epub 2022 Sep 7. Annu Rev Pathol. 2023. PMID: 36070562 Free PMC article. Review.
-
Endothelial NOX5 Expression Modulates Thermogenesis and Lipolysis in Mice Fed with a High-Fat Diet and 3T3-L1 Adipocytes through an Interleukin-6 Dependent Mechanism.Antioxidants (Basel). 2021 Dec 24;11(1):30. doi: 10.3390/antiox11010030. Antioxidants (Basel). 2021. PMID: 35052534 Free PMC article.
-
CHRNA2: a new paradigm in beige thermoregulation and metabolism.Trends Cell Biol. 2022 Jun;32(6):479-489. doi: 10.1016/j.tcb.2021.11.009. Epub 2021 Dec 21. Trends Cell Biol. 2022. PMID: 34952750 Free PMC article. Review.
-
CCE and EODF as two distinct non-shivering thermogenesis models inducing weight loss.Pflugers Arch. 2023 Aug;475(8):961-974. doi: 10.1007/s00424-023-02827-7. Epub 2023 Jun 15. Pflugers Arch. 2023. PMID: 37386129
-
A multi-dimensional view of context-dependent G protein-coupled receptor function.Biochem Soc Trans. 2023 Feb 27;51(1):13-20. doi: 10.1042/BST20210650. Biochem Soc Trans. 2023. PMID: 36688421 Free PMC article. Review.
References
-
- Ahmed K., Tunaru S., Tang C., Müller M., Gille A., Sassmann A., Hanson J., Offermanns S. An autocrine lactate loop mediates insulin-dependent inhibition of lipolysis through GPR81. Cell Metab. 2010;11:311–319. - PubMed
-
- Bachman E.S., Dhillon H., Zhang C.-Y., Cinti S., Bianco A.C., Kobilka B.K., Lowell B.B. betaAR signaling required for diet-induced thermogenesis and obesity resistance. Science. 2002;297:843–845. - PubMed
-
- Balaz M., Becker A.S., Balazova L., Straub L., Müller J., Gashi G., Maushart C.I., Sun W., Dong H., Moser C. Inhibition of Mevalonate Pathway Prevents Adipocyte Browning in Mice and Men by Affecting Protein Prenylation. Cell Metab. 2019;29:901–916.e8. - PubMed
Publication types
MeSH terms
Substances
Grants and funding
LinkOut - more resources
Full Text Sources
Other Literature Sources
Molecular Biology Databases