The multifaceted nature of antimicrobial peptides: current synthetic chemistry approaches and future directions
- PMID: 34042120
- PMCID: PMC8689412
- DOI: 10.1039/d0cs00729c
The multifaceted nature of antimicrobial peptides: current synthetic chemistry approaches and future directions
Erratum in
-
Correction: The multifaceted nature of antimicrobial peptides: current synthetic chemistry approaches and future directions.Chem Soc Rev. 2022 Jan 24;51(2):792. doi: 10.1039/d1cs90109e. Chem Soc Rev. 2022. PMID: 34935805 Free PMC article.
Abstract
Bacterial infections caused by 'superbugs' are increasing globally, and conventional antibiotics are becoming less effective against these bacteria, such that we risk entering a post-antibiotic era. In recent years, antimicrobial peptides (AMPs) have gained significant attention for their clinical potential as a new class of antibiotics to combat antimicrobial resistance. In this review, we discuss several facets of AMPs including their diversity, physicochemical properties, mechanisms of action, and effects of environmental factors on these features. This review outlines various chemical synthetic strategies that have been applied to develop novel AMPs, including chemical modifications of existing peptides, semi-synthesis, and computer-aided design. We will also highlight novel AMP structures, including hybrids, antimicrobial dendrimers and polypeptides, peptidomimetics, and AMP-drug conjugates and consider recent developments in their chemical synthesis.
Conflict of interest statement
There are no conflicts to declare.
Figures
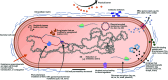
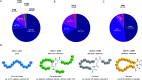
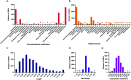
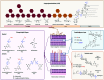
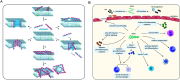
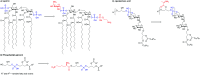
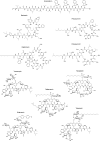
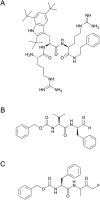
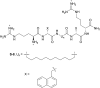
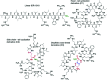
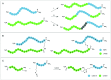
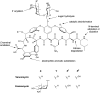
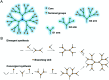
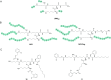
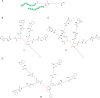
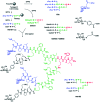
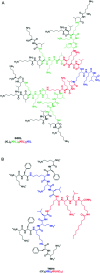
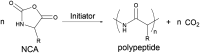
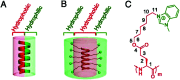
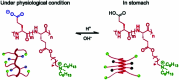
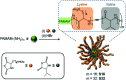
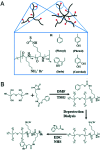
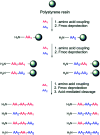
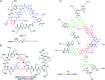
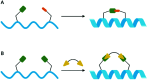
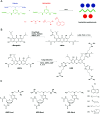
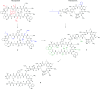
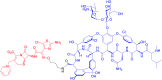
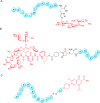
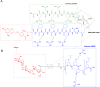
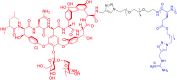
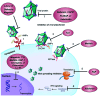
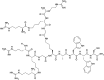

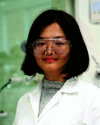
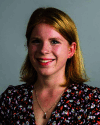
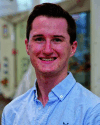
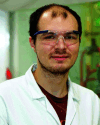
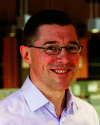
Similar articles
-
Towards the Development of Synthetic Antibiotics: Designs Inspired by Natural Antimicrobial Peptides.Curr Med Chem. 2016;23(41):4610-4624. doi: 10.2174/0929867323666160825162435. Curr Med Chem. 2016. PMID: 27570165 Review.
-
AApeptides as a new class of antimicrobial agents.Org Biomol Chem. 2013 Jul 14;11(26):4283-90. doi: 10.1039/c3ob40444g. Epub 2013 May 31. Org Biomol Chem. 2013. PMID: 23722277 Review.
-
Synergism between Host Defence Peptides and Antibiotics Against Bacterial Infections.Curr Top Med Chem. 2020;20(14):1238-1263. doi: 10.2174/1568026620666200303122626. Curr Top Med Chem. 2020. PMID: 32124698 Review.
-
Advancements in peptide-based antimicrobials: A possible option for emerging drug-resistant infections.Adv Colloid Interface Sci. 2024 Nov;333:103282. doi: 10.1016/j.cis.2024.103282. Epub 2024 Sep 6. Adv Colloid Interface Sci. 2024. PMID: 39276418 Review.
-
Recent Developments in Antimicrobial-Peptide-Conjugated Gold Nanoparticles.Bioconjug Chem. 2017 Nov 15;28(11):2673-2686. doi: 10.1021/acs.bioconjchem.7b00368. Epub 2017 Sep 26. Bioconjug Chem. 2017. PMID: 28892365 Review.
Cited by
-
Damage-associated molecular patterns in vitiligo: igniter fuse from oxidative stress to melanocyte loss.Redox Rep. 2022 Dec;27(1):193-199. doi: 10.1080/13510002.2022.2123864. Redox Rep. 2022. PMID: 36154894 Free PMC article. Review.
-
Antimicrobial Peptides Targeting Streptococcus mutans: Current Research on Design, Screening and Efficacy.Curr Microbiol. 2023 Nov 25;81(1):18. doi: 10.1007/s00284-023-03540-5. Curr Microbiol. 2023. PMID: 38007405 Review.
-
Prokaryotic Expression, Purification, and Biological Properties of a Novel Bioactive Protein (PFAP-1) from Pinctada fucata.Mar Drugs. 2024 Jul 27;22(8):345. doi: 10.3390/md22080345. Mar Drugs. 2024. PMID: 39195461 Free PMC article.
-
Mapping of the Lipid-Binding Regions of the Antifungal Protein NFAP2 by Exploiting Model Membranes.J Chem Inf Model. 2024 Aug 26;64(16):6557-6569. doi: 10.1021/acs.jcim.4c00229. Epub 2024 Aug 16. J Chem Inf Model. 2024. PMID: 39150323 Free PMC article.
-
Effects of Dimerization, Dendrimerization, and Chirality in p-BthTX-I Peptide Analogs on the Antibacterial Activity and Enzymatic Inhibition of the SARS-CoV-2 PLpro Protein.Pharmaceutics. 2023 Jan 28;15(2):436. doi: 10.3390/pharmaceutics15020436. Pharmaceutics. 2023. PMID: 36839758 Free PMC article.
References
-
- Walsh C., Antibiotics: actions, origins, resistance, 1st edn, ASM Press, 2003
-
- Patrick G. L., An Introduction to Medicinal Chemistry, 4th edn, Oxford University Press Inc., New York, 2009
-
- 21st Model List of Essential Medicines, World Health Organisation, https://www.who.int/groups/expert-committee-on-selection-and-use-of-esse... (accessed 12 November 2020)
Publication types
MeSH terms
Substances
LinkOut - more resources
Full Text Sources
Other Literature Sources