Synthetic living machines: A new window on life
- PMID: 34041452
- PMCID: PMC8141884
- DOI: 10.1016/j.isci.2021.102505
Synthetic living machines: A new window on life
Abstract
Increased control of biological growth and form is an essential gateway to transformative medical advances. Repairing of birth defects, restoring lost or damaged organs, normalizing tumors, all depend on understanding how cells cooperate to make specific, functional large-scale structures. Despite advances in molecular genetics, significant gaps remain in our understanding of the meso-scale rules of morphogenesis. An engineering approach to this problem is the creation of novel synthetic living forms, greatly extending available model systems beyond evolved plant and animal lineages. Here, we review recent advances in the emerging field of synthetic morphogenesis, the bioengineering of novel multicellular living bodies. Emphasizing emergent self-organization, tissue-level guided self-assembly, and active functionality, this work is the essential next generation of synthetic biology. Aside from useful living machines for specific functions, the rational design and analysis of new, coherent anatomies will greatly increase our understanding of foundational questions in evolutionary developmental and cell biology.
Keywords: bioengineering; developmental biology; synthetic biology.
© 2021 The Author(s).
Conflict of interest statement
M.L. is a co-inventor on U.S. Application 63/136,564 submitted by Tufts University that covers some engineered multicellular organisms. M.R.E. is a co-inventor on US9677085B2 and Application WO2019237124 and PCT/US2020/045926, which are related to engineering multicellular systems.
Figures
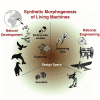
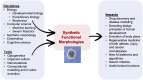
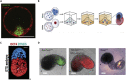
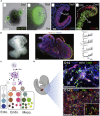

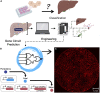
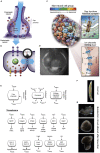
Similar articles
-
Life, death, and self: Fundamental questions of primitive cognition viewed through the lens of body plasticity and synthetic organisms.Biochem Biophys Res Commun. 2021 Jul 30;564:114-133. doi: 10.1016/j.bbrc.2020.10.077. Epub 2020 Nov 6. Biochem Biophys Res Commun. 2021. PMID: 33162026 Review.
-
Macromolecular crowding: chemistry and physics meet biology (Ascona, Switzerland, 10-14 June 2012).Phys Biol. 2013 Aug;10(4):040301. doi: 10.1088/1478-3975/10/4/040301. Epub 2013 Aug 2. Phys Biol. 2013. PMID: 23912807
-
Synthetic biology meets tissue engineering.Biochem Soc Trans. 2016 Jun 15;44(3):696-701. doi: 10.1042/BST20150289. Biochem Soc Trans. 2016. PMID: 27284030 Free PMC article. Review.
-
The mismeasure of machine: Synthetic biology and the trouble with engineering metaphors.Stud Hist Philos Biol Biomed Sci. 2013 Dec;44(4 Pt B):660-8. doi: 10.1016/j.shpsc.2013.05.013. Epub 2013 Jun 18. Stud Hist Philos Biol Biomed Sci. 2013. PMID: 23790452
-
Synthetic developmental biology: build and control multicellular systems.Curr Opin Chem Biol. 2019 Oct;52:9-15. doi: 10.1016/j.cbpa.2019.04.006. Epub 2019 May 15. Curr Opin Chem Biol. 2019. PMID: 31102790 Review.
Cited by
-
Behaviorist approaches to investigating memory and learning: A primer for synthetic biology and bioengineering.Commun Integr Biol. 2021 Dec 14;14(1):230-247. doi: 10.1080/19420889.2021.2005863. eCollection 2021. Commun Integr Biol. 2021. PMID: 34925687 Free PMC article. Review.
-
Motile Living Biobots Self-Construct from Adult Human Somatic Progenitor Seed Cells.Adv Sci (Weinh). 2024 Jan;11(4):e2303575. doi: 10.1002/advs.202303575. Epub 2023 Nov 30. Adv Sci (Weinh). 2024. PMID: 38032125 Free PMC article.
-
Decellularization in Tissue Engineering and Regenerative Medicine: Evaluation, Modification, and Application Methods.Front Bioeng Biotechnol. 2022 Apr 25;10:805299. doi: 10.3389/fbioe.2022.805299. eCollection 2022. Front Bioeng Biotechnol. 2022. PMID: 35547166 Free PMC article. Review.
-
Cell-Free Gene Expression in Bioprinted Fluidic Networks.ACS Synth Biol. 2024 Aug 16;13(8):2447-2456. doi: 10.1021/acssynbio.4c00187. Epub 2024 Jul 23. ACS Synth Biol. 2024. PMID: 39042670 Free PMC article.
-
Spatial biology of Ising-like synthetic genetic networks.BMC Biol. 2023 Sep 4;21(1):185. doi: 10.1186/s12915-023-01681-4. BMC Biol. 2023. PMID: 37667283 Free PMC article.
References
Publication types
Grants and funding
LinkOut - more resources
Full Text Sources
Other Literature Sources