Interactions between capsid and viral RNA regulate Chikungunya virus translation in a host-specific manner
- PMID: 34023723
- PMCID: PMC8206026
- DOI: 10.1016/j.virol.2021.04.009
Interactions between capsid and viral RNA regulate Chikungunya virus translation in a host-specific manner
Abstract
Alphaviruses are positive sense, RNA viruses commonly transmitted by an arthropod vector to a mammalian or avian host. In recent years, a number of the Alphavirus members have reemerged as public health concerns. Transmission from mosquito vector to vertebrate hosts requires an understanding of the interaction between the virus and both vertebrate and insect hosts to develop rational intervention strategies. The current study uncovers a novel role for capsid protein during Chikungunya virus replication whereby the interaction with viral RNA in the E1 coding region regulates protein synthesis processes early in infection. Studies done in both the mammalian and mosquito cells indicate that interactions between viral RNA and capsid protein have functional consequences that are host species specific. Our data support a vertebrate-specific role for capsid:vRNA interaction in temporally regulating viral translation in a manner dependent on the PI3K-AKT-mTOR pathway.
Keywords: Alphavirus; Capsid protein; PI3K-AKT-mTOR; Translation.
Copyright © 2021 Elsevier Inc. All rights reserved.
Conflict of interest statement
Declaration of interests
The authors declare that they have no known competing financial interests or personal relationships that could have appeared to influence the work reported in this paper.
Figures
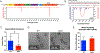
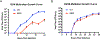
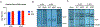
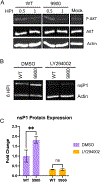
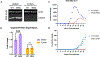
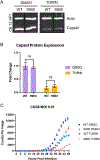
Similar articles
-
Design and Use of Chikungunya Virus Replication Templates Utilizing Mammalian and Mosquito RNA Polymerase I-Mediated Transcription.J Virol. 2019 Aug 28;93(18):e00794-19. doi: 10.1128/JVI.00794-19. Print 2019 Sep 15. J Virol. 2019. PMID: 31217251 Free PMC article.
-
Role for the phosphatidylinositol 3-kinase-Akt-TOR pathway during sindbis virus replication in arthropods.J Virol. 2012 Apr;86(7):3595-604. doi: 10.1128/JVI.06625-11. Epub 2012 Jan 18. J Virol. 2012. PMID: 22258238 Free PMC article.
-
Mutation of the N-Terminal Region of Chikungunya Virus Capsid Protein: Implications for Vaccine Design.mBio. 2017 Feb 21;8(1):e01970-16. doi: 10.1128/mBio.01970-16. mBio. 2017. PMID: 28223458 Free PMC article.
-
Tropism of the Chikungunya Virus.Viruses. 2019 Feb 20;11(2):175. doi: 10.3390/v11020175. Viruses. 2019. PMID: 30791607 Free PMC article. Review.
-
Chikungunya virus and its mosquito vectors.Vector Borne Zoonotic Dis. 2015 Apr;15(4):231-40. doi: 10.1089/vbz.2014.1745. Epub 2015 Feb 12. Vector Borne Zoonotic Dis. 2015. PMID: 25674945 Review.
Cited by
-
Compositional complementarity between genomic RNA and coat proteins in positive-sense single-stranded RNA viruses.Nucleic Acids Res. 2022 Apr 22;50(7):4054-4067. doi: 10.1093/nar/gkac202. Nucleic Acids Res. 2022. PMID: 35357492 Free PMC article.
-
Distinct chikungunya virus polymerase palm subdomains contribute to viral protein accumulation and virion production.PLoS Pathog. 2024 Oct 14;20(10):e1011972. doi: 10.1371/journal.ppat.1011972. eCollection 2024 Oct. PLoS Pathog. 2024. PMID: 39401243 Free PMC article.
References
-
- Ahola T and Merits A (2016). “Functions of Chikungunya Virus Nonstructural Proteins.” Chikungunya Virus: Advances in Biology, Pathogenesis, and Treatment: 75–98.
-
- Arias-Goeta C, Moutailler S, Mousson L, Zouache K, Thiberge JM, Caro V, Rougeon F and Failloux AB (2014). “Chikungunya virus adaptation to a mosquito vector correlates with only few point mutations in the viral envelope glycoprotein.” Infect Genet Evol 24: 116–126. - PubMed
-
- Broeckel R, Sarkar S, May NA, Totonchy J, Kreklywich CN, Smith P, Graves L, DeFilippis VR, Heise MT, Morrison TE, Moorman N and Streblow DN (2019). “Src Family Kinase Inhibitors Block Translation of Alphavirus Subgenomic mRNAs.” Antimicrobial Agents and Chemotherapy 63(4): e02325–02318. - PMC - PubMed
Publication types
MeSH terms
Substances
Grants and funding
LinkOut - more resources
Full Text Sources
Other Literature Sources
Medical
Miscellaneous