LMO3 reprograms visceral adipocyte metabolism during obesity
- PMID: 34018016
- PMCID: PMC8313462
- DOI: 10.1007/s00109-021-02089-9
LMO3 reprograms visceral adipocyte metabolism during obesity
Abstract
Obesity and body fat distribution are important risk factors for the development of type 2 diabetes and metabolic syndrome. Evidence has accumulated that this risk is related to intrinsic differences in behavior of adipocytes in different fat depots. We recently identified LIM domain only 3 (LMO3) in human mature visceral adipocytes; however, its function in these cells is currently unknown. The aim of this study was to determine the potential involvement of LMO3-dependent pathways in the modulation of key functions of mature adipocytes during obesity. Based on a recently engineered hybrid rAAV serotype Rec2 shown to efficiently transduce both brown adipose tissue (BAT) and white adipose tissue (WAT), we delivered YFP or Lmo3 to epididymal WAT (eWAT) of C57Bl6/J mice on a high-fat diet (HFD). The effects of eWAT transduction on metabolic parameters were evaluated 10 weeks later. To further define the role of LMO3 in insulin-stimulated glucose uptake, insulin signaling, adipocyte bioenergetics, as well as endocrine function, experiments were conducted in 3T3-L1 adipocytes and newly differentiated human primary mature adipocytes, engineered for transient gain or loss of LMO3 expression, respectively. AAV transduction of eWAT results in strong and stable Lmo3 expression specifically in the adipocyte fraction over a course of 10 weeks with HFD feeding. LMO3 expression in eWAT significantly improved insulin sensitivity and healthy visceral adipose tissue expansion in diet-induced obesity, paralleled by increased serum adiponectin. In vitro, LMO3 expression in 3T3-L1 adipocytes increased PPARγ transcriptional activity, insulin-stimulated GLUT4 translocation and glucose uptake, as well as mitochondrial oxidative capacity in addition to fatty acid oxidation. Mechanistically, LMO3 induced the PPARγ coregulator Ncoa1, which was required for LMO3 to enhance glucose uptake and mitochondrial oxidative gene expression. In human mature adipocytes, LMO3 overexpression promoted, while silencing of LMO3 suppressed mitochondrial oxidative capacity. LMO3 expression in visceral adipose tissue regulates multiple genes that preserve adipose tissue functionality during obesity, such as glucose metabolism, insulin sensitivity, mitochondrial function, and adiponectin secretion. Together with increased PPARγ activity and Ncoa1 expression, these gene expression changes promote insulin-induced GLUT4 translocation, glucose uptake in addition to increased mitochondrial oxidative capacity, limiting HFD-induced adipose dysfunction. These data add LMO3 as a novel regulator improving visceral adipose tissue function during obesity. KEY MESSAGES: LMO3 increases beneficial visceral adipose tissue expansion and insulin sensitivity in vivo. LMO3 increases glucose uptake and oxidative mitochondrial activity in adipocytes. LMO3 increases nuclear coactivator 1 (Ncoa1). LMO3-enhanced glucose uptake and mitochondrial gene expression requires Ncoa1.
Keywords: LMO3; Obesity; Visceral adipose tissue.
© 2021. The Author(s).
Conflict of interest statement
The authors declare no competing interests.
Figures
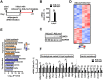
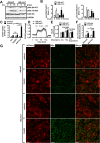
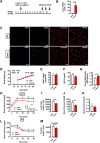
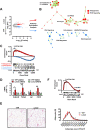
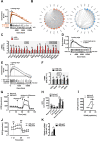
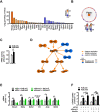
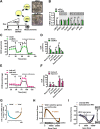
Similar articles
-
Pinch Loss Ameliorates Obesity, Glucose Intolerance, and Fatty Liver by Modulating Adipocyte Apoptosis in Mice.Diabetes. 2021 Nov;70(11):2492-2505. doi: 10.2337/db21-0392. Epub 2021 Aug 11. Diabetes. 2021. PMID: 34380695
-
Artemisia extracts activate PPARγ, promote adipogenesis, and enhance insulin sensitivity in adipose tissue of obese mice.Nutrition. 2014 Jul-Aug;30(7-8 Suppl):S31-6. doi: 10.1016/j.nut.2014.02.013. Epub 2014 Mar 12. Nutrition. 2014. PMID: 24985103 Free PMC article.
-
Artemisia scoparia enhances adipocyte development and endocrine function in vitro and enhances insulin action in vivo.PLoS One. 2014 Jun 10;9(6):e98897. doi: 10.1371/journal.pone.0098897. eCollection 2014. PLoS One. 2014. PMID: 24915004 Free PMC article.
-
Adipocyte Mitochondria: Deciphering Energetic Functions across Fat Depots in Obesity and Type 2 Diabetes.Int J Mol Sci. 2024 Jun 18;25(12):6681. doi: 10.3390/ijms25126681. Int J Mol Sci. 2024. PMID: 38928386 Free PMC article. Review.
-
The neutral amino acid transporter SLC7A10 in adipose tissue, obesity and insulin resistance.Front Cell Dev Biol. 2022 Sep 12;10:974338. doi: 10.3389/fcell.2022.974338. eCollection 2022. Front Cell Dev Biol. 2022. PMID: 36172277 Free PMC article. Review.
Cited by
-
Precision medicine: toward restoring fat with gene therapy in inherited lipodystrophy.Gene Ther. 2024 Nov;31(11-12):560-562. doi: 10.1038/s41434-024-00489-3. Epub 2024 Sep 24. Gene Ther. 2024. PMID: 39317737 No abstract available.
-
LMO3 downregulation in PCa: A prospective biomarker associated with immune infiltration.Front Genet. 2022 Sep 19;13:945151. doi: 10.3389/fgene.2022.945151. eCollection 2022. Front Genet. 2022. PMID: 36199576 Free PMC article.
-
Whole-Genome Sequencing Data Reveal New Loci Affecting Milk Production in German Black Pied Cattle (DSN).Genes (Basel). 2023 Feb 25;14(3):581. doi: 10.3390/genes14030581. Genes (Basel). 2023. PMID: 36980854 Free PMC article.
-
New Mediators in the Crosstalk between Different Adipose Tissues.Int J Mol Sci. 2024 Apr 25;25(9):4659. doi: 10.3390/ijms25094659. Int J Mol Sci. 2024. PMID: 38731880 Free PMC article. Review.
-
Impact of Maternal Mediterranean-Type Diet Adherence on Microbiota Composition and Epigenetic Programming of Offspring.Nutrients. 2023 Dec 22;16(1):47. doi: 10.3390/nu16010047. Nutrients. 2023. PMID: 38201877 Free PMC article.
References
-
- Lindroos J, Husa J, Mitterer G, Haschemi A, Rauscher S, Haas R, Gröger M, Loewe R, Kohrgruber N, Schrögendorfer KF, Prager G, Beck H, Pospisilik JA, Zeyda M, Stulnig TM, Patsch W, Wagner O, Esterbauer H, Bilban M. Human but not mouse adipogenesis is critically dependent on LMO3. Cell Metab. 2013;18(1):62–74. doi: 10.1016/j.cmet.2013.05.020. - DOI - PMC - PubMed
-
- He W, Barak Y, Hevener A, Olson P, Liao D, le J, Nelson M, Ong E, Olefsky JM, Evans RM. Adipose-specific peroxisome proliferator-activated receptor gamma knockout causes insulin resistance in fat and liver but not in muscle. Proc Natl Acad Sci U S A. 2003;100(26):15712–15717. doi: 10.1073/pnas.2536828100. - DOI - PMC - PubMed
Publication types
MeSH terms
Substances
Grants and funding
LinkOut - more resources
Full Text Sources
Other Literature Sources
Medical
Molecular Biology Databases
Research Materials
Miscellaneous