Water-oriented magnetic anisotropy transition
- PMID: 33980833
- PMCID: PMC8115317
- DOI: 10.1038/s41467-021-23057-4
Water-oriented magnetic anisotropy transition
Abstract
Water reorientation is essential in a wide range of chemical and biological processes. However, the effects of such reorientation through rotation around the metal-oxygen bond on the chemical and physical properties of the resulting complex are usually ignored. Most studies focus on the donor property of water as a recognized σ donor-type ligand rather than a participant in the π interaction. Although a theoretical approach to study water-rotation effects on the functionality of a complex has recently been conducted, it has not been experimentally demonstrated. In this study, we determine that the magnetic anisotropy of a Co(II) complex can be effectively controlled by the slight rotation of coordinating water ligands, which is achieved by a two-step structural phase transition. When the water molecule is rotated by 21.2 ± 0.2° around the Co-O bond, the directional magnetic susceptibility of the single crystal changes by approximately 30% along the a-axis due to the rotation of the magnetic anisotropy axis through the modification of the π interaction between cobalt(II) and the water ligand. The theoretical calculations further support the hypothesis that the reorientation of water molecules is a key factor contributing to the magnetic anisotropy transition of this complex.
Conflict of interest statement
The authors declare no competing interests.
Figures
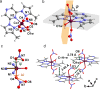
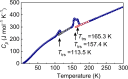
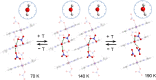
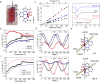
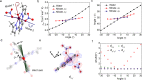
Similar articles
-
Anisotropic Change in the Magnetic Susceptibility of a Dynamic Single Crystal of a Cobalt(II) Complex.Angew Chem Int Ed Engl. 2017 Jan 16;56(3):717-721. doi: 10.1002/anie.201606165. Epub 2016 Dec 9. Angew Chem Int Ed Engl. 2017. PMID: 27936289
-
A structure-based analysis of the vibrational spectra of nitrosyl ligands in transition-metal coordination complexes and clusters.Spectrochim Acta A Mol Biomol Spectrosc. 2011 Jan;78(1):7-28. doi: 10.1016/j.saa.2010.08.001. Epub 2010 Aug 17. Spectrochim Acta A Mol Biomol Spectrosc. 2011. PMID: 21123107
-
Ferromagnetic coupling and magnetic anisotropy in oxalato-bridged trinuclear chromium(III)-cobalt(II) complexes with aromatic diimine ligands.Dalton Trans. 2010 Mar 7;39(9):2350-8. doi: 10.1039/b915546e. Epub 2009 Dec 23. Dalton Trans. 2010. PMID: 20162209
-
Ultrafast 2D IR anisotropy of water reveals reorientation during hydrogen-bond switching.J Chem Phys. 2011 Aug 7;135(5):054509. doi: 10.1063/1.3623008. J Chem Phys. 2011. PMID: 21823714
-
Role of Coordination Number and Geometry in Controlling the Magnetic Anisotropy in FeII , CoII , and NiII Single-Ion Magnets.Chemistry. 2020 Nov 6;26(62):14036-14058. doi: 10.1002/chem.202003211. Epub 2020 Oct 12. Chemistry. 2020. PMID: 32729641 Review.
References
-
- Sessoli R, Gatteschi D, Caneschi A, Novak MA. Magnetic bistability in a metal-ion cluster. Nature. 1993;365:141–143. doi: 10.1038/365141a0. - DOI
LinkOut - more resources
Full Text Sources
Other Literature Sources
Research Materials
Miscellaneous