Diversity and Functions of Type II Topoisomerases
- PMID: 33959387
- PMCID: PMC8084294
- DOI: 10.32607/actanaturae.11058
Diversity and Functions of Type II Topoisomerases
Abstract
The DNA double helix provides a simple and elegant way to store and copy genetic information. However, the processes requiring the DNA helix strands separation, such as transcription and replication, induce a topological side-effect - supercoiling of the molecule. Topoisomerases comprise a specific group of enzymes that disentangle the topological challenges associated with DNA supercoiling. They relax DNA supercoils and resolve catenanes and knots. Here, we review the catalytic cycles, evolution, diversity, and functional roles of type II topoisomerases in organisms from all domains of life, as well as viruses and other mobile genetic elements.
Keywords: DNA segregation; decatenation; replication; spatial chromosome organization; supercoiling; topoisomerases; transcription.
Copyright ® 2021 National Research University Higher School of Economics.
Figures
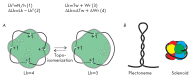
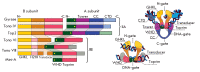
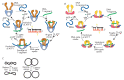
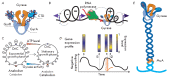
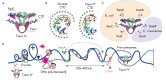
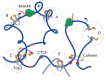
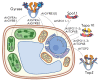
Similar articles
-
DNA-Topology Simplification by Topoisomerases.Molecules. 2021 Jun 3;26(11):3375. doi: 10.3390/molecules26113375. Molecules. 2021. PMID: 34204901 Free PMC article. Review.
-
The regulation of DNA supercoiling across evolution.Protein Sci. 2021 Oct;30(10):2042-2056. doi: 10.1002/pro.4171. Epub 2021 Aug 23. Protein Sci. 2021. PMID: 34398513 Free PMC article. Review.
-
DNA-Stimulated Liquid-Liquid phase separation by eukaryotic topoisomerase ii modulates catalytic function.Elife. 2022 Nov 7;11:e81786. doi: 10.7554/eLife.81786. Elife. 2022. PMID: 36342377 Free PMC article.
-
Mechanisms of separation of the complementary strands of DNA during replication.Genetica. 1999;106(1-2):131-40. doi: 10.1023/a:1003749416449. Genetica. 1999. PMID: 10710719 Review.
-
[DNA supercoiling and topoisomerases in Escherichia coli].Rev Latinoam Microbiol. 1995 Jul-Sep;37(3):291-304. Rev Latinoam Microbiol. 1995. PMID: 8850348 Review. Spanish.
Cited by
-
The role of prokaryotic argonautes in resistance to type II topoisomerases poison ciprofloxacin.Biochem Soc Trans. 2024 Oct 30;52(5):2157-2166. doi: 10.1042/BST20240094. Biochem Soc Trans. 2024. PMID: 39446311 Free PMC article. Review.
-
Structure-function analysis of the ATPase domain of African swine fever virus topoisomerase.mBio. 2024 Apr 10;15(4):e0308623. doi: 10.1128/mbio.03086-23. Epub 2024 Feb 27. mBio. 2024. PMID: 38411066 Free PMC article.
-
Dynamics of chromosome organization in a minimal bacterial cell.Front Cell Dev Biol. 2023 Aug 9;11:1214962. doi: 10.3389/fcell.2023.1214962. eCollection 2023. Front Cell Dev Biol. 2023. PMID: 37621774 Free PMC article.
-
CRISPR/Cas9 Essential Gene Editing in Drosophila.Acta Naturae. 2023 Apr-Jun;15(2):70-74. doi: 10.32607/actanaturae.11874. Acta Naturae. 2023. PMID: 37538801 Free PMC article.
-
Base excision repair and double strand break repair cooperate to modulate the formation of unrepaired double strand breaks in mouse brain.Nat Commun. 2024 Sep 4;15(1):7726. doi: 10.1038/s41467-024-51906-5. Nat Commun. 2024. PMID: 39231940 Free PMC article.
References
-
- Mirkin S. Encyclopedia of Life Sciences. Chichester: John Wiley & Sons Ltd., 2001. 2001.
-
- Bates A., Maxwell A. DNA topology. Second Ed. Oxford: Oxford University Press, 2005. 2005.
-
- Maxwell A., Bush N.G., Evans-Roberts K., EcoSal Plus. 2015;6(2):1–34. - PubMed
LinkOut - more resources
Full Text Sources
Other Literature Sources