CCR2 Regulates Vaccine-Induced Mucosal T-Cell Memory to Influenza A Virus
- PMID: 33952647
- PMCID: PMC8274608
- DOI: 10.1128/JVI.00530-21
CCR2 Regulates Vaccine-Induced Mucosal T-Cell Memory to Influenza A Virus
Abstract
Elicitation of lung tissue-resident memory CD8 T cells (TRMs) is a goal of T cell-based vaccines against respiratory viral pathogens, such as influenza A virus (IAV). C-C chemokine receptor type 2 (CCR2)-dependent monocyte trafficking plays an essential role in the establishment of CD8 TRMs in lungs of IAV-infected mice. Here, we used a combination adjuvant-based subunit vaccine strategy that evokes multifaceted (TC1/TC17/TH1/TH17) IAV nucleoprotein-specific lung TRMs to determine whether CCR2 and monocyte infiltration are essential for vaccine-induced TRM development and protective immunity to IAV in lungs. Following intranasal vaccination, neutrophils, monocytes, conventional dendritic cells (DCs), and monocyte-derived dendritic cells internalized and processed vaccine antigen in lungs. We found that basic leucine zipper ATF-like transcription factor 3 (BATF3)-dependent DCs were essential for eliciting T cell responses, but CCR2 deficiency enhanced the differentiation of CD127hi, KLRG-1lo, OX40+ve CD62L+ve, and mucosally imprinted CD69+ve CD103+ve effector and memory CD8 T cells in lungs and airways of vaccinated mice. Mechanistically, increased development of lung TRMs induced by CCR2 deficiency was linked to dampened expression of T-bet but not altered TCF-1 levels or T cell receptor signaling in CD8 T cells. T1/T17 functional programming, parenchymal localization of CD8/CD4 effector and memory T cells, recall T cell responses, and protective immunity to a lethal IAV infection were unaffected in CCR2-deficient mice. Taken together, we identified a negative regulatory role for CCR2 and monocyte trafficking in mucosal imprinting and differentiation of vaccine-induced TRMs. Mechanistic insights from this study may aid the development of T-cell-based vaccines against respiratory viral pathogens, including IAV and severe acute respiratory syndrome coronavirus 2 (SARS-CoV-2). IMPORTANCE While antibody-based immunity to influenza A virus (IAV) is type and subtype specific, lung- and airway-resident memory T cells that recognize conserved epitopes in the internal viral proteins are known to provide heterosubtypic immunity. Hence, broadly protective IAV vaccines need to elicit robust T cell memory in the respiratory tract. We have developed a combination adjuvant-based IAV nucleoprotein vaccine that elicits strong CD4 and CD8 T cell memory in lungs and protects against H1N1 and H5N1 strains of IAV. In this study, we examined the mechanisms that control vaccine-induced protective memory T cells in the respiratory tract. We found that trafficking of monocytes into lungs might limit the development of antiviral lung-resident memory T cells following intranasal vaccination. These findings suggest that strategies that limit monocyte infiltration can potentiate vaccine-induced frontline T-cell immunity to respiratory viruses, such as IAV and SARS-CoV-2.
Keywords: CD8 T cells; adjuvants; influenza vaccines; memory; mucosal adjuvants; subunit vaccines; tissue-resident memory.
Figures
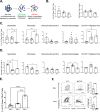
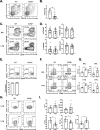
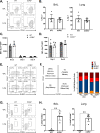
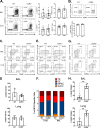
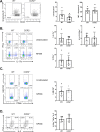
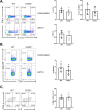
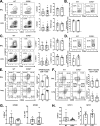
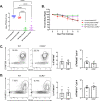
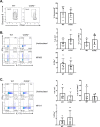
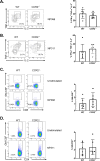
Update of
-
CCR2 Regulates Vaccine-Induced Mucosal T-Cell Memory to Influenza A Virus.bioRxiv [Preprint]. 2021 Mar 25:2021.03.24.436901. doi: 10.1101/2021.03.24.436901. bioRxiv. 2021. Update in: J Virol. 2021 Jul 12;95(15):e0053021. doi: 10.1128/JVI.00530-21 PMID: 33791695 Free PMC article. Updated. Preprint.
Similar articles
-
CCR2 Regulates Vaccine-Induced Mucosal T-Cell Memory to Influenza A Virus.bioRxiv [Preprint]. 2021 Mar 25:2021.03.24.436901. doi: 10.1101/2021.03.24.436901. bioRxiv. 2021. Update in: J Virol. 2021 Jul 12;95(15):e0053021. doi: 10.1128/JVI.00530-21 PMID: 33791695 Free PMC article. Updated. Preprint.
-
Programming Multifaceted Pulmonary T Cell Immunity by Combination Adjuvants.Cell Rep Med. 2020 Sep 22;1(6):100095. doi: 10.1016/j.xcrm.2020.100095. Cell Rep Med. 2020. PMID: 32984856 Free PMC article.
-
Effective Respiratory CD8 T-Cell Immunity to Influenza Virus Induced by Intranasal Carbomer-Lecithin-Adjuvanted Non-replicating Vaccines.PLoS Pathog. 2016 Dec 20;12(12):e1006064. doi: 10.1371/journal.ppat.1006064. eCollection 2016 Dec. PLoS Pathog. 2016. PMID: 27997610 Free PMC article.
-
Potentiating Lung Mucosal Immunity Through Intranasal Vaccination.Front Immunol. 2021 Dec 14;12:808527. doi: 10.3389/fimmu.2021.808527. eCollection 2021. Front Immunol. 2021. PMID: 34970279 Free PMC article. Review.
-
Influenza-Specific Lung-Resident Memory CD8+ T Cells.Cold Spring Harb Perspect Biol. 2021 Feb 1;13(2):a037978. doi: 10.1101/cshperspect.a037978. Cold Spring Harb Perspect Biol. 2021. PMID: 33288540 Free PMC article. Review.
Cited by
-
Vaccine adjuvants to engage the cross-presentation pathway.Front Immunol. 2022 Aug 1;13:940047. doi: 10.3389/fimmu.2022.940047. eCollection 2022. Front Immunol. 2022. PMID: 35979365 Free PMC article. Review.
-
Single-cell analysis reveals dysregulated inflammatory response in peripheral blood immunity in patients with acute respiratory distress syndrome.Front Cell Dev Biol. 2023 May 22;11:1199122. doi: 10.3389/fcell.2023.1199122. eCollection 2023. Front Cell Dev Biol. 2023. PMID: 37283946 Free PMC article.
-
Methionine enkephalin(MENK) upregulated memory T cells in anti-influenza response.BMC Immunol. 2023 Oct 12;24(1):38. doi: 10.1186/s12865-023-00573-0. BMC Immunol. 2023. PMID: 37828468 Free PMC article.
-
Multimodal profiling of lung granulomas in macaques reveals cellular correlates of tuberculosis control.Immunity. 2022 May 10;55(5):827-846.e10. doi: 10.1016/j.immuni.2022.04.004. Epub 2022 Apr 27. Immunity. 2022. PMID: 35483355 Free PMC article.
References
Publication types
MeSH terms
Substances
Grants and funding
LinkOut - more resources
Full Text Sources
Other Literature Sources
Medical
Molecular Biology Databases
Research Materials
Miscellaneous