Complex Mechanisms of Antimony Genotoxicity in Budding Yeast Involves Replication and Topoisomerase I-Associated DNA Lesions, Telomere Dysfunction and Inhibition of DNA Repair
- PMID: 33925940
- PMCID: PMC8123508
- DOI: 10.3390/ijms22094510
Complex Mechanisms of Antimony Genotoxicity in Budding Yeast Involves Replication and Topoisomerase I-Associated DNA Lesions, Telomere Dysfunction and Inhibition of DNA Repair
Abstract
Antimony is a toxic metalloid with poorly understood mechanisms of toxicity and uncertain carcinogenic properties. By using a combination of genetic, biochemical and DNA damage assays, we investigated the genotoxic potential of trivalent antimony in the model organism Saccharomyces cerevisiae. We found that low doses of Sb(III) generate various forms of DNA damage including replication and topoisomerase I-dependent DNA lesions as well as oxidative stress and replication-independent DNA breaks accompanied by activation of DNA damage checkpoints and formation of recombination repair centers. At higher concentrations of Sb(III), moderately increased oxidative DNA damage is also observed. Consistently, base excision, DNA damage tolerance and homologous recombination repair pathways contribute to Sb(III) tolerance. In addition, we provided evidence suggesting that Sb(III) causes telomere dysfunction. Finally, we showed that Sb(III) negatively effects repair of double-strand DNA breaks and distorts actin and microtubule cytoskeleton. In sum, our results indicate that Sb(III) exhibits a significant genotoxic activity in budding yeast.
Keywords: DNA damage; DNA repair; antimony; cell cycle checkpoints; genotoxicity.
Conflict of interest statement
The authors declare no conflict of interest.
Figures
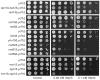
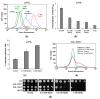
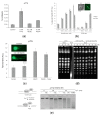
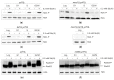
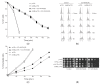
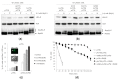
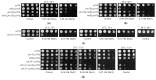
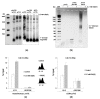
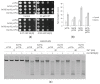
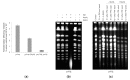
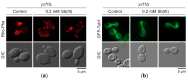
Similar articles
-
Oxidative stress and replication-independent DNA breakage induced by arsenic in Saccharomyces cerevisiae.PLoS Genet. 2013;9(7):e1003640. doi: 10.1371/journal.pgen.1003640. Epub 2013 Jul 25. PLoS Genet. 2013. PMID: 23935510 Free PMC article.
-
Mode of action assessment of the genotoxic properties of antimony and its compounds evaluated in the ToxTracker assay.Mutat Res Genet Toxicol Environ Mutagen. 2021 May;865:503333. doi: 10.1016/j.mrgentox.2021.503333. Epub 2021 Feb 15. Mutat Res Genet Toxicol Environ Mutagen. 2021. PMID: 33865539
-
Investigations on the role of base excision repair and non-homologous end-joining pathways in sodium selenite-induced toxicity and mutagenicity in Saccharomyces cerevisiae.Mutagenesis. 2010 Mar;25(2):155-62. doi: 10.1093/mutage/gep056. Epub 2009 Dec 2. Mutagenesis. 2010. PMID: 19955329
-
Regulation of homologous recombination at telomeres in budding yeast.FEBS Lett. 2010 Sep 10;584(17):3696-702. doi: 10.1016/j.febslet.2010.05.037. Epub 2010 May 24. FEBS Lett. 2010. PMID: 20580716 Review.
-
Break-induced replication and recombinational telomere elongation in yeast.Annu Rev Biochem. 2006;75:111-35. doi: 10.1146/annurev.biochem.74.082803.133234. Annu Rev Biochem. 2006. PMID: 16756487 Review.
Cited by
-
Multilevel Regulation of Membrane Proteins in Response to Metal and Metalloid Stress: A Lesson from Yeast.Int J Mol Sci. 2024 Apr 18;25(8):4450. doi: 10.3390/ijms25084450. Int J Mol Sci. 2024. PMID: 38674035 Free PMC article. Review.
-
Mechanisms of genotoxicity and proteotoxicity induced by the metalloids arsenic and antimony.Cell Mol Life Sci. 2023 Oct 30;80(11):342. doi: 10.1007/s00018-023-04992-5. Cell Mol Life Sci. 2023. PMID: 37904059 Free PMC article. Review.
-
Interplay between the oxidation process and cytotoxic effects of antimonene nanomaterials.Nanoscale. 2024 May 23;16(20):9754-9769. doi: 10.1039/d4nr00532e. Nanoscale. 2024. PMID: 38625086 Free PMC article.
References
-
- Filella M., Belzile N., Chen Y.W. Antimony in the environment: A review focused on natural waters: I. Occurrence. Earth Sci. Rev. 2002;57:125–176. doi: 10.1016/S0012-8252(01)00070-8. - DOI
-
- Wang X., He M., Xie J., Xi J., Lu X. Heavy metal pollution of the world largest antimony mine-affected agricultural soils in Hunan province (China) J. Soils Sediments. 2010;10:827–837. doi: 10.1007/s11368-010-0196-4. - DOI
MeSH terms
Substances
Grants and funding
LinkOut - more resources
Full Text Sources
Other Literature Sources
Molecular Biology Databases