Annexins A2, A6 and Fetuin-A Affect the Process of Mineralization in Vesicles Derived from Human Osteoblastic hFOB 1.19 and Osteosarcoma Saos-2 Cells
- PMID: 33924370
- PMCID: PMC8069967
- DOI: 10.3390/ijms22083993
Annexins A2, A6 and Fetuin-A Affect the Process of Mineralization in Vesicles Derived from Human Osteoblastic hFOB 1.19 and Osteosarcoma Saos-2 Cells
Abstract
The mineralization process is initiated by osteoblasts and chondrocytes during intramembranous and endochondral ossifications, respectively. Both types of cells release matrix vesicles (MVs), which accumulate Pi and Ca2+ and form apatites in their lumen. Tissue non-specific alkaline phosphatase (TNAP), a mineralization marker, is highly enriched in MVs, in which it removes inorganic pyrophosphate (PPi), an inhibitor of apatite formation. MVs then bud from the microvilli of mature osteoblasts or hypertrophic chondrocytes and, thanks to the action of the acto-myosin cortex, become released to the extracellular matrix (ECM), where they bind to collagen fibers and propagate mineral growth. In this report, we compared the mineralization ability of human fetal osteoblastic cell line (hFOB 1.19 cells) with that of osteosarcoma cell line (Saos-2 cells). Both types of cells were able to mineralize in an osteogenic medium containing ascorbic acid and beta glycerophosphate. The composition of calcium and phosphate compounds in cytoplasmic vesicles was distinct from that in extracellular vesicles (mostly MVs) released after collagenase-digestion. Apatites were identified only in MVs derived from Saos-2 cells, while MVs from hFOB 1.19 cells contained amorphous calcium phosphate complexes. In addition, AnxA6 and AnxA2 (nucleators of mineralization) increased mineralization in the sub-membrane region in strongly mineralizing Saos-2 osteosarcoma, where they co-localized with TNAP, whereas in less mineralizing hFOB 1.19 osteoblasts, AnxA6, and AnxA2 co-localizations with TNAP were less visible in the membrane. We also observed a reduction in the level of fetuin-A (FetuA), an inhibitor of mineralization in ECM, following treatment with TNAP and Ca channels inhibitors, especially in osteosarcoma cells. Moreover, a fraction of FetuA was translocated from the cytoplasm towards the plasma membrane during the stimulation of Saos-2 cells, while this displacement was less pronounced in stimulated hFOB 19 cells. In summary, osteosarcoma Saos-2 cells had a better ability to mineralize than osteoblastic hFOB 1.19 cells. The formation of apatites was observed in Saos-2 cells, while only complexes of calcium and phosphate were identified in hFOB 1.19 cells. This was also evidenced by a more pronounced accumulation of AnxA2, AnxA6, FetuA in the plasma membrane, where they were partly co-localized with TNAP in Saos-2 cells, in comparison to hFOB 1.19 cells. This suggests that both activators (AnxA2, AnxA6) and inhibitors (FetuA) of mineralization were recruited to the membrane and co-localized with TNAP to take part in the process of mineralization.
Keywords: Saos-2 osteosarcoma cells; annexins; fetuin-A; hFOB 1.19 osteoblastic cells; matrix vesicles; mineralization.
Conflict of interest statement
The authors declare no conflict of interest.
Figures
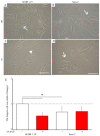
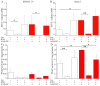
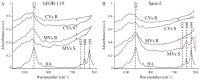
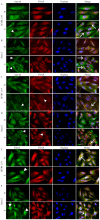
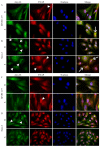
Similar articles
-
Apigenin Modulates AnxA6- and TNAP-Mediated Osteoblast Mineralization.Int J Mol Sci. 2022 Oct 29;23(21):13179. doi: 10.3390/ijms232113179. Int J Mol Sci. 2022. PMID: 36361965 Free PMC article.
-
Src and ROCK Kinases Differentially Regulate Mineralization of Human Osteosarcoma Saos-2 Cells.Int J Mol Sci. 2019 Jun 12;20(12):2872. doi: 10.3390/ijms20122872. Int J Mol Sci. 2019. PMID: 31212828 Free PMC article.
-
Characteristics of minerals in vesicles produced by human osteoblasts hFOB 1.19 and osteosarcoma Saos-2 cells stimulated for mineralization.J Inorg Biochem. 2017 Jun;171:100-107. doi: 10.1016/j.jinorgbio.2017.03.006. Epub 2017 Mar 29. J Inorg Biochem. 2017. PMID: 28380345
-
The role of phosphatases in the initiation of skeletal mineralization.Calcif Tissue Int. 2013 Oct;93(4):299-306. doi: 10.1007/s00223-012-9672-8. Epub 2012 Nov 27. Calcif Tissue Int. 2013. PMID: 23183786 Free PMC article. Review.
-
The roles of annexins and alkaline phosphatase in mineralization process.Acta Biochim Pol. 2003;50(4):1019-38. Acta Biochim Pol. 2003. PMID: 14739992 Review.
Cited by
-
Extracellular vesicles in tumorigenesis, metastasis, chemotherapy resistance and intercellular communication in osteosarcoma.Bioengineered. 2023 Dec;14(1):113-128. doi: 10.1080/21655979.2022.2161711. Bioengineered. 2023. PMID: 37377390 Free PMC article. Review.
-
The Physiological and Pathological Role of Tissue Nonspecific Alkaline Phosphatase beyond Mineralization.Biomolecules. 2021 Oct 21;11(11):1564. doi: 10.3390/biom11111564. Biomolecules. 2021. PMID: 34827562 Free PMC article. Review.
-
Systematic analysis of RNA-binding proteins identifies targetable therapeutic vulnerabilities in osteosarcoma.Nat Commun. 2024 Apr 1;15(1):2810. doi: 10.1038/s41467-024-47031-y. Nat Commun. 2024. PMID: 38561347 Free PMC article.
-
Apigenin Modulates AnxA6- and TNAP-Mediated Osteoblast Mineralization.Int J Mol Sci. 2022 Oct 29;23(21):13179. doi: 10.3390/ijms232113179. Int J Mol Sci. 2022. PMID: 36361965 Free PMC article.
-
ANXA6: a key molecular player in cancer progression and drug resistance.Discov Oncol. 2023 May 2;14(1):53. doi: 10.1007/s12672-023-00662-x. Discov Oncol. 2023. PMID: 37129645 Free PMC article. Review.
References
-
- Bottini M., Mebarek S., Anderson K.L., Strzelecka-Kiliszek A., Bozycki L., Simão A.M.S., Bolean M., Ciancaglini P., Pikula J.B., Pikula S., et al. Matrix vesicles from chondrocytes and osteoblasts: Their biogenesis, properties, functions and biomimetic models. Biochim. Biophys. Acta (BBA) Gen. Subj. 2018;1862:532–546. doi: 10.1016/j.bbagen.2017.11.005. - DOI - PMC - PubMed
MeSH terms
Substances
LinkOut - more resources
Full Text Sources
Other Literature Sources
Molecular Biology Databases
Research Materials
Miscellaneous