Human Adipose Stem Cells (hASCs) Grown on Biodegradable Microcarriers in Serum- and Xeno-Free Medium Preserve Their Undifferentiated Status
- PMID: 33923488
- PMCID: PMC8167760
- DOI: 10.3390/jfb12020025
Human Adipose Stem Cells (hASCs) Grown on Biodegradable Microcarriers in Serum- and Xeno-Free Medium Preserve Their Undifferentiated Status
Abstract
Human adipose stem cells (hASCs) are promising candidates for cell-based therapies, but they need to be efficiently expanded in vitro as they cannot be harvested in sufficient quantities. Recently, dynamic bioreactor systems operated with microcarriers achieved considerable high cell densities. Thus, they are a viable alternative to static planar cultivation systems to obtain high numbers of clinical-grade hASCs. Nevertheless, the production of considerable biomass in a short time must not be achieved to the detriment of the cells' quality. To facilitate the scalable expansion of hASC, we have developed a new serum- and xeno-free medium (UrSuppe) and a biodegradable microcarrier (BR44). In this study, we investigated whether the culture of hASCs in defined serum-free conditions on microcarriers (3D) or on planar (2D) cell culture vessels may influence the expression of some marker genes linked with the immature degree or the differentiated status of the cells. Furthermore, we investigated whether the biomaterials, which form our biodegradable MCs, may affect cell behavior and differentiation. The results confirmed that the quality and the undifferentiated status of the hASCs are very well preserved when they grow on BR44 MCs in defined serum-free conditions. Indeed, the ASCs showed a gene expression profile more compatible with an undifferentiated status than the same cells grown under standard planar conditions.
Keywords: UrSuppe; biodegradable microcarrier (MC); biomaterial; human adipose stem cells (hASCs); serum- and xeno-free cell culture medium.
Conflict of interest statement
Matias Lindner and Michele Müller are Sferalp SA employees who manufacture the microcarrier
Figures
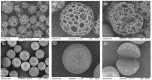
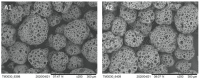
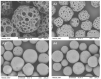
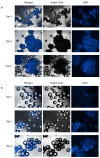
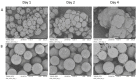
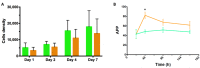
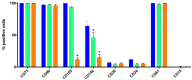
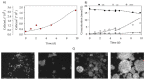
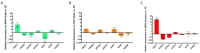
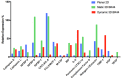
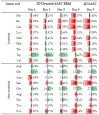
Similar articles
-
Emerging Trends in Biodegradable Microcarriers for Therapeutic Applications.Polymers (Basel). 2023 Mar 16;15(6):1487. doi: 10.3390/polym15061487. Polymers (Basel). 2023. PMID: 36987266 Free PMC article. Review.
-
Chemically Defined, Xeno-Free Expansion of Human Mesenchymal Stem Cells (hMSCs) on Benchtop-Scale Using a Stirred Single-Use Bioreactor.Methods Mol Biol. 2022;2436:83-111. doi: 10.1007/7651_2021_426. Methods Mol Biol. 2022. PMID: 34611815
-
An Approach towards a GMP Compliant In-Vitro Expansion of Human Adipose Stem Cells for Autologous Therapies.Bioengineering (Basel). 2020 Jul 20;7(3):77. doi: 10.3390/bioengineering7030077. Bioengineering (Basel). 2020. PMID: 32698363 Free PMC article.
-
Chemically Defined Xeno- and Serum-Free Cell Culture Medium to Grow Human Adipose Stem Cells.Cells. 2021 Feb 22;10(2):466. doi: 10.3390/cells10020466. Cells. 2021. PMID: 33671568 Free PMC article.
-
Microcarrier-based platforms for in vitro expansion and differentiation of human pluripotent stem cells in bioreactor culture systems.J Biotechnol. 2016 Sep 20;234:71-82. doi: 10.1016/j.jbiotec.2016.07.023. Epub 2016 Jul 29. J Biotechnol. 2016. PMID: 27480342 Review.
Cited by
-
Cellular In Vitro Responses Induced by Human Mesenchymal Stem/Stromal Cell-Derived Extracellular Vesicles Obtained from Suspension Culture.Int J Mol Sci. 2024 Jul 11;25(14):7605. doi: 10.3390/ijms25147605. Int J Mol Sci. 2024. PMID: 39062847 Free PMC article.
-
Emerging Trends in Biodegradable Microcarriers for Therapeutic Applications.Polymers (Basel). 2023 Mar 16;15(6):1487. doi: 10.3390/polym15061487. Polymers (Basel). 2023. PMID: 36987266 Free PMC article. Review.
-
Enhancement of therapeutic potential of mesenchymal stem cell by IGF-1 delivery in PLGA microspheres for tissue regeneration.Regen Ther. 2024 Mar 23;27:112-119. doi: 10.1016/j.reth.2024.03.004. eCollection 2024 Dec. Regen Ther. 2024. PMID: 38550913 Free PMC article.
-
Chemically Defined, Xeno-Free Expansion of Human Mesenchymal Stem Cells (hMSCs) on Benchtop-Scale Using a Stirred Single-Use Bioreactor.Methods Mol Biol. 2022;2436:83-111. doi: 10.1007/7651_2021_426. Methods Mol Biol. 2022. PMID: 34611815
-
A Review of the Use of Microparticles for Cartilage Tissue Engineering.Int J Mol Sci. 2021 Sep 24;22(19):10292. doi: 10.3390/ijms221910292. Int J Mol Sci. 2021. PMID: 34638629 Free PMC article. Review.
References
-
- Bourin P., Bunnell B.A., Casteilla L., Dominici M., Katz A.J., March K.L., Redl H., Rubin J.P., Yoshimura K., Gimble J.M. Stromal cells from the adipose tissue-derived stromal vascular fraction and culture expanded adipose tissue-derived stromal/stem cells: A joint statement of the International Federation for Adipose Therapeutics and Science (IFATS) and the International Society for Cellular Therapy (ISCT) Cytotherapy. 2013;15:641–648. doi: 10.1016/j.jcyt.2013.02.006. - DOI - PMC - PubMed
Grants and funding
LinkOut - more resources
Full Text Sources
Other Literature Sources