Bacteriophage-Based Biosensing of Pseudomonas aeruginosa: An Integrated Approach for the Putative Real-Time Detection of Multi-Drug-Resistant Strains
- PMID: 33921071
- PMCID: PMC8071457
- DOI: 10.3390/bios11040124
Bacteriophage-Based Biosensing of Pseudomonas aeruginosa: An Integrated Approach for the Putative Real-Time Detection of Multi-Drug-Resistant Strains
Abstract
During the last decennium, it has become widely accepted that ubiquitous bacterial viruses, or bacteriophages, exert enormous influences on our planet's biosphere, killing between 4-50% of the daily produced bacteria and constituting the largest genetic diversity pool on our planet. Currently, bacterial infections linked to healthcare services are widespread, which, when associated with the increasing surge of antibiotic-resistant microorganisms, play a major role in patient morbidity and mortality. In this scenario, Pseudomonas aeruginosa alone is responsible for ca. 13-15% of all hospital-acquired infections. The pathogen P. aeruginosa is an opportunistic one, being endowed with metabolic versatility and high (both intrinsic and acquired) resistance to antibiotics. Bacteriophages (or phages) have been recognized as a tool with high potential for the detection of bacterial infections since these metabolically inert entities specifically attach to, and lyse, bacterial host cells, thus, allowing confirmation of the presence of viable cells. In the research effort described herein, three different phages with broad lytic spectrum capable of infecting P. aeruginosa were isolated from environmental sources. The isolated phages were elected on the basis of their ability to form clear and distinctive plaques, which is a hallmark characteristic of virulent phages. Next, their structural and functional stabilization was achieved via entrapment within the matrix of porous alginate, biopolymeric, and bio-reactive, chromogenic hydrogels aiming at their use as sensitive matrices producing both color changes and/or light emissions evolving from a reaction with (released) cytoplasmic moieties, as a bio-detection kit for P. aeruginosa cells. Full physicochemical and biological characterization of the isolated bacteriophages was the subject of a previous research paper.
Keywords: Pseudomonas aeruginosa; bacterial biosensing; bacteriophage particles; bio-reactive polymeric matrix; chromogenic/bioluminescent bio-hydrogel; immobilization and structural/functional stabilization.
Conflict of interest statement
The authors declare no conflict of interest.
Figures
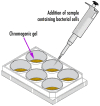
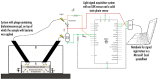
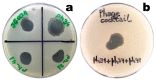
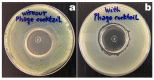
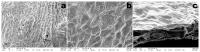
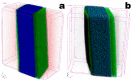
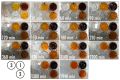
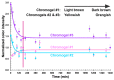
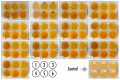
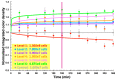
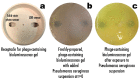
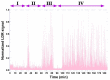
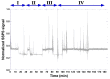
Similar articles
-
Structural and functional stabilization of phage particles in carbohydrate matrices for bacterial biosensing.Enzyme Microb Technol. 2013 Jun 10;53(1):55-69. doi: 10.1016/j.enzmictec.2013.03.001. Epub 2013 Apr 15. Enzyme Microb Technol. 2013. PMID: 23683705
-
Carbohydrate hydrogels with stabilized phage particles for bacterial biosensing: bacterium diffusion studies.Appl Biochem Biotechnol. 2014 Feb;172(3):1194-214. doi: 10.1007/s12010-013-0579-2. Epub 2013 Oct 23. Appl Biochem Biotechnol. 2014. PMID: 24146368
-
Isolation and Characterization of Lytic Bacteriophage Against Multi-drug Resistant Pseudomonas aeruginosa.J Nepal Health Res Counc. 2022 Mar 13;19(4):717-724. doi: 10.33314/jnhrc.v19i04.3837. J Nepal Health Res Counc. 2022. PMID: 35615828
-
Antibiotic resistance in Pseudomonas aeruginosa - Mechanisms, epidemiology and evolution.Drug Resist Updat. 2019 May;44:100640. doi: 10.1016/j.drup.2019.07.002. Epub 2019 Jul 19. Drug Resist Updat. 2019. PMID: 31492517 Review.
-
Bacteriophages of Pseudomonas aeruginosa: long-term prospects for use in phage therapy.Adv Virus Res. 2014;88:227-78. doi: 10.1016/B978-0-12-800098-4.00005-2. Adv Virus Res. 2014. PMID: 24373314 Review.
Cited by
-
Bacteriophage-based bioassays: an expected paradigm shift in microbial diagnostics.Future Microbiol. 2024 Jun 12;19(9):811-824. doi: 10.2217/fmb-2023-0246. Epub 2024 Jun 20. Future Microbiol. 2024. PMID: 38900594 Free PMC article. Review.
-
An Edible Biopolymeric Microcapsular Wrapping Integrating Lytic Bacteriophage Particles for Salmonella enterica: Potential for Integration into Poultry Feed.Antibiotics (Basel). 2023 May 31;12(6):988. doi: 10.3390/antibiotics12060988. Antibiotics (Basel). 2023. PMID: 37370307 Free PMC article.
-
A Synergistic Dual-Channel Sensor for Ultrasensitive Detection of Pseudomonas aeruginosa by DNA Nanostructure and G-Quadruplex.Biosensors (Basel). 2022 Dec 26;13(1):24. doi: 10.3390/bios13010024. Biosensors (Basel). 2022. PMID: 36671859 Free PMC article.
-
Isolation and Molecular Characterization of Two Novel Lytic Bacteriophages for the Biocontrol of Escherichia coli in Uterine Infections: In Vitro and Ex Vivo Preliminary Studies in Veterinary Medicine.Pharmaceutics. 2022 Oct 30;14(11):2344. doi: 10.3390/pharmaceutics14112344. Pharmaceutics. 2022. PMID: 36365162 Free PMC article.
-
Measurement of Low Concentration of Micro-Plastics by Detection of Bioaffinity-Induced Particle Retention Using Surface Plasmon Resonance Biosensors.Biosensors (Basel). 2021 Jul 3;11(7):219. doi: 10.3390/bios11070219. Biosensors (Basel). 2021. PMID: 34356691 Free PMC article.
References
-
- Rios A.C., Vila M.M.D.C., Lima R., Del Fiol F.S., Tubino M., Teixeira J.A., Balcão V.M. Structural and functional stabilization of bacteriophage particles within the aqueous core of a W/O/W multiple emulsion: A potential biotherapeutic system for the inhalational treatment of bacterial pneumonia. Process Biochem. 2018;64:177–192. doi: 10.1016/j.procbio.2017.09.022. - DOI
-
- Caselli D., Cesaro S., Ziino O., Zanazzo G., Manicone R., Livadiotti S., Cellini M., Frenos S., Milano G.M., Cappelli B., et al. Multidrug resistant Pseudomonas aeruginosa infection in children undergoing chemotherapy and hematopoietic stem cell transplantation. Haematologica. 2010;95:1612–1615. doi: 10.3324/haematol.2009.020867. - DOI - PMC - PubMed
MeSH terms
Substances
Grants and funding
- 2016/08884-3/Fundação de Amparo à Pesquisa do Estado de São Paulo
- 2016/12234-4/Fundação de Amparo à Pesquisa do Estado de São Paulo
- 2018/05522-9/Fundação de Amparo à Pesquisa do Estado de São Paulo
- 306113/2014-7/Conselho Nacional de Desenvolvimento Científico e Tecnológico
- 308208/2017-0/Conselho Nacional de Desenvolvimento Científico e Tecnológico
LinkOut - more resources
Full Text Sources
Other Literature Sources