DDX3 depletion represses translation of mRNAs with complex 5' UTRs
- PMID: 33905506
- PMCID: PMC8136831
- DOI: 10.1093/nar/gkab287
DDX3 depletion represses translation of mRNAs with complex 5' UTRs
Abstract
DDX3 is an RNA chaperone of the DEAD-box family that regulates translation. Ded1, the yeast ortholog of DDX3, is a global regulator of translation, whereas DDX3 is thought to preferentially affect a subset of mRNAs. However, the set of mRNAs that are regulated by DDX3 are unknown, along with the relationship between DDX3 binding and activity. Here, we use ribosome profiling, RNA-seq, and PAR-CLIP to define the set of mRNAs that are regulated by DDX3 in human cells. We find that while DDX3 binds highly expressed mRNAs, depletion of DDX3 particularly affects the translation of a small subset of the transcriptome. We further find that DDX3 binds a site on helix 16 of the human ribosomal rRNA, placing it immediately adjacent to the mRNA entry channel. Translation changes caused by depleting DDX3 levels or expressing an inactive point mutation are different, consistent with different association of these genetic variant types with disease. Taken together, this work defines the subset of the transcriptome that is responsive to DDX3 inhibition, with relevance for basic biology and disease states where DDX3 is altered.
© The Author(s) 2021. Published by Oxford University Press on behalf of Nucleic Acids Research.
Figures
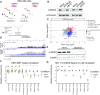
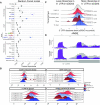
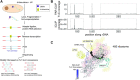
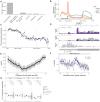
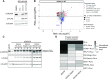
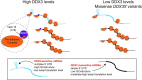
Similar articles
-
DDX3 regulates cell growth through translational control of cyclin E1.Mol Cell Biol. 2010 Nov;30(22):5444-53. doi: 10.1128/MCB.00560-10. Epub 2010 Sep 13. Mol Cell Biol. 2010. PMID: 20837705 Free PMC article.
-
DEAD-box protein DDX3 associates with eIF4F to promote translation of selected mRNAs.EMBO J. 2012 Sep 12;31(18):3745-56. doi: 10.1038/emboj.2012.220. Epub 2012 Aug 7. EMBO J. 2012. PMID: 22872150 Free PMC article.
-
Genome-wide analysis of translational efficiency reveals distinct but overlapping functions of yeast DEAD-box RNA helicases Ded1 and eIF4A.Genome Res. 2015 Aug;25(8):1196-205. doi: 10.1101/gr.191601.115. Epub 2015 Jun 29. Genome Res. 2015. PMID: 26122911 Free PMC article.
-
The translational landscape as regulated by the RNA helicase DDX3.BMB Rep. 2022 Mar;55(3):125-135. doi: 10.5483/BMBRep.2022.55.3.188. BMB Rep. 2022. PMID: 35236544 Free PMC article. Review.
-
Targeting RNA helicases in cancer: The translation trap.Biochim Biophys Acta Rev Cancer. 2017 Dec;1868(2):510-520. doi: 10.1016/j.bbcan.2017.09.006. Epub 2017 Sep 28. Biochim Biophys Acta Rev Cancer. 2017. PMID: 28965870 Free PMC article. Review.
Cited by
-
The human DEAD-box helicase DDX3X as a regulator of mRNA translation.Front Cell Dev Biol. 2022 Oct 25;10:1033684. doi: 10.3389/fcell.2022.1033684. eCollection 2022. Front Cell Dev Biol. 2022. PMID: 36393867 Free PMC article. Review.
-
Characterization and optimization of 5´ untranslated region containing poly-adenine tracts in Kluyveromyces marxianus using machine-learning model.Microb Cell Fact. 2024 Jan 3;23(1):7. doi: 10.1186/s12934-023-02271-3. Microb Cell Fact. 2024. PMID: 38172836 Free PMC article.
-
Aberrant cortical development is driven by impaired cell cycle and translational control in a DDX3X syndrome model.Elife. 2022 Jun 28;11:e78203. doi: 10.7554/eLife.78203. Elife. 2022. PMID: 35762573 Free PMC article.
-
RNA Helicases as Shadow Modulators of Cell Cycle Progression.Int J Mol Sci. 2021 Mar 15;22(6):2984. doi: 10.3390/ijms22062984. Int J Mol Sci. 2021. PMID: 33804185 Free PMC article. Review.
-
Ceftriaxone exerts antitumor effects in MYCN-driven retinoblastoma and neuroblastoma by targeting DDX3X for translation repression.Mol Oncol. 2024 Apr;18(4):918-938. doi: 10.1002/1878-0261.13553. Epub 2023 Nov 27. Mol Oncol. 2024. PMID: 37975412 Free PMC article.
References
-
- Sharma D., Jankowsky E.. The Ded1/DDX3 subfamily of DEAD-box RNA helicases. Crit. Rev. Biochem. Mol. Biol. 2014; 49:343–360. - PubMed
Publication types
MeSH terms
Substances
Grants and funding
LinkOut - more resources
Full Text Sources
Other Literature Sources
Molecular Biology Databases
Research Materials