Reconstitution of GABA, Glycine and Glutamate Transporters
- PMID: 33905037
- PMCID: PMC8763731
- DOI: 10.1007/s11064-021-03331-z
Reconstitution of GABA, Glycine and Glutamate Transporters
Abstract
In contrast to water soluble enzymes which can be purified and studied while in solution, studies of solute carrier (transporter) proteins require both that the protein of interest is situated in a phospholipid membrane and that this membrane forms a closed compartment. An additional challenge to the study of transporter proteins has been that the transport depends on the transmembrane electrochemical gradients. Baruch I. Kanner understood this early on and first developed techniques for studying plasma membrane vesicles. This advanced the field in that the experimenter could control the electrochemical gradients. Kanner, however, did not stop there, but started to solubilize the membranes so that the transporter proteins were taken out of their natural environment. In order to study them, Kanner then had to find a way to reconstitute them (reinsert them into phospholipid membranes). The scope of the present review is both to describe the reconstitution method in full detail as that has never been done, and also to reveal the scientific impact that this method has had. Kanner's later work is not reviewed here although that also deserves a review because it too has had a huge impact.
Keywords: GABA transporter; Glutamate transporter; Glycine transporter; Liposomes; Reconstitution; Scientific history.
© 2021. The Author(s).
Conflict of interest statement
The authors declare that they have no conflict of interest.
Figures
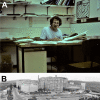
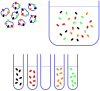
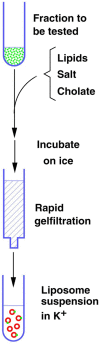
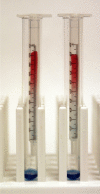
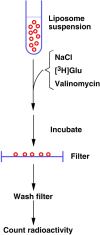
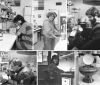
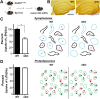
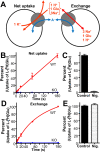
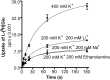
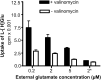
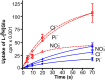
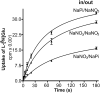
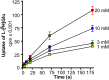
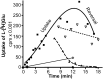
Similar articles
-
Functional reconstitution of the gamma-aminobutyric acid transporter from synaptic vesicles using artificial ion gradients.Biochemistry. 1991 Dec 24;30(51):11795-800. doi: 10.1021/bi00115a009. Biochemistry. 1991. PMID: 1684290
-
Vesicular neurotransmitter transporter expression in developing postnatal rodent retina: GABA and glycine precede glutamate.J Neurosci. 2003 Jan 15;23(2):518-29. doi: 10.1523/JNEUROSCI.23-02-00518.2003. J Neurosci. 2003. PMID: 12533612 Free PMC article.
-
Activation of gamma-aminobutyric acid GAT-1 transporters on glutamatergic terminals of mouse spinal cord mediates glutamate release through anion channels and by transporter reversal.J Neurosci Res. 2005 May 1;80(3):424-33. doi: 10.1002/jnr.20437. J Neurosci Res. 2005. PMID: 15789377
-
Structure, function and brain localization of neurotransmitter transporters.J Exp Biol. 1994 Nov;196:283-95. doi: 10.1242/jeb.196.1.283. J Exp Biol. 1994. PMID: 7823028 Review.
-
Glial transporters for glutamate, glycine, and GABA: II. GABA transporters.J Neurosci Res. 2001 Mar 15;63(6):461-8. doi: 10.1002/jnr.1040. J Neurosci Res. 2001. PMID: 11241581 Review.
Cited by
-
Interactions Involving Glycine and Other Amino Acid Neurotransmitters: Focus on Transporter-Mediated Regulation of Release and Glycine-Glutamate Crosstalk.Biomedicines. 2024 Jul 8;12(7):1518. doi: 10.3390/biomedicines12071518. Biomedicines. 2024. PMID: 39062091 Free PMC article. Review.
References
Publication types
MeSH terms
Substances
Grants and funding
LinkOut - more resources
Full Text Sources