Heterogeneity in transmissibility and shedding SARS-CoV-2 via droplets and aerosols
- PMID: 33861198
- PMCID: PMC8139838
- DOI: 10.7554/eLife.65774
Heterogeneity in transmissibility and shedding SARS-CoV-2 via droplets and aerosols
Abstract
Background: Which virological factors mediate overdispersion in the transmissibility of emerging viruses remains a long-standing question in infectious disease epidemiology.
Methods: Here, we use systematic review to develop a comprehensive dataset of respiratory viral loads (rVLs) of SARS-CoV-2, SARS-CoV-1 and influenza A(H1N1)pdm09. We then comparatively meta-analyze the data and model individual infectiousness by shedding viable virus via respiratory droplets and aerosols.
Results: The analyses indicate heterogeneity in rVL as an intrinsic virological factor facilitating greater overdispersion for SARS-CoV-2 in the COVID-19 pandemic than A(H1N1)pdm09 in the 2009 influenza pandemic. For COVID-19, case heterogeneity remains broad throughout the infectious period, including for pediatric and asymptomatic infections. Hence, many COVID-19 cases inherently present minimal transmission risk, whereas highly infectious individuals shed tens to thousands of SARS-CoV-2 virions/min via droplets and aerosols while breathing, talking and singing. Coughing increases the contagiousness, especially in close contact, of symptomatic cases relative to asymptomatic ones. Infectiousness tends to be elevated between 1 and 5 days post-symptom onset.
Conclusions: Intrinsic case variation in rVL facilitates overdispersion in the transmissibility of emerging respiratory viruses. Our findings present considerations for disease control in the COVID-19 pandemic as well as future outbreaks of novel viruses.
Funding: Natural Sciences and Engineering Research Council of Canada (NSERC) Discovery Grant program, NSERC Senior Industrial Research Chair program and the Toronto COVID-19 Action Fund.
Keywords: COVID-19; emerging pathogens; epidemiology; global health; infectious disease; influenza; microbiology; none; overdispersion; superspreading.
Plain language summary
To understand how viruses spread scientists look at two things. One is – on average – how many other people each infected person spreads the virus to. The other is how much variability there is in the number of people each person with the virus infects. Some viruses like the 2009 influenza H1N1, a new strain of influenza that caused a pandemic beginning in 2009, spread pretty uniformly, with many people with the virus infecting around two other people. Other viruses like SARS-CoV-2, the one that causes COVID-19, are more variable. About 10 to 20% of people with COVID-19 cause 80% of subsequent infections – which may lead to so-called superspreading events – while 60-75% of people with COVID-19 infect no one else. Learning more about these differences can help public health officials create better ways to curb the spread of the virus. Chen et al. show that differences in the concentration of virus particles in the respiratory tract may help to explain why superspreaders play such a big role in transmitting SARS-CoV-2, but not the 2009 influenza H1N1 virus. Chen et al. reviewed and extracted data from studies that have collected how much virus is present in people infected with either SARS-CoV-2, a similar virus called SARS-CoV-1 that caused the SARS outbreak in 2003, or with 2009 influenza H1N1. Chen et al. found that as the variability in the concentration of the virus in the airways increased, so did the variability in the number of people each person with the virus infects. Chen et al. further used mathematical models to estimate how many virus particles individuals with each infection would expel via droplets or aerosols, based on the differences in virus concentrations from their analyses. The models showed that most people with COVID-19 infect no one because they expel little – if any – infectious SARS-CoV-2 when they talk, breathe, sing or cough. Highly infectious individuals on the other hand have high concentrations of the virus in their airways, particularly the first few days after developing symptoms, and can expel tens to thousands of infectious virus particles per minute. By contrast, a greater proportion of people with 2009 influenza H1N1 were potentially infectious but tended to expel relatively little infectious virus when the talk, sing, breathe or cough. These results help explain why superspreaders play such a key role in the ongoing pandemic. This information suggests that to stop this virus from spreading it is important to limit crowd sizes, shorten the duration of visits or gatherings, maintain social distancing, talk in low volumes around others, wear masks, and hold gatherings in well-ventilated settings. In addition, contact tracing can prioritize the contacts of people with high concentrations of virus in their airways.
© 2021, Chen et al.
Conflict of interest statement
PC, NB, ZP, MK, DF, FG No competing interests declared
Figures
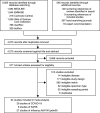
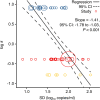
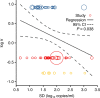
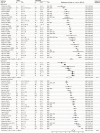
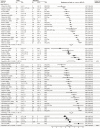
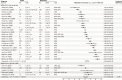
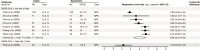
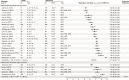
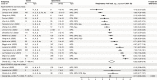
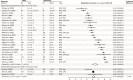
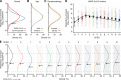
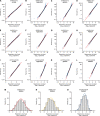
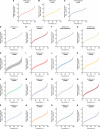
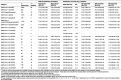
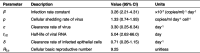
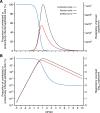
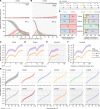
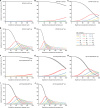
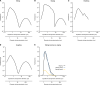
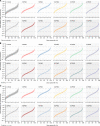
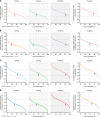
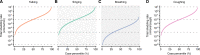
Similar articles
-
Physical interventions to interrupt or reduce the spread of respiratory viruses.Cochrane Database Syst Rev. 2020 Nov 20;11(11):CD006207. doi: 10.1002/14651858.CD006207.pub5. Cochrane Database Syst Rev. 2020. Update in: Cochrane Database Syst Rev. 2023 Jan 30;1:CD006207. doi: 10.1002/14651858.CD006207.pub6 PMID: 33215698 Free PMC article. Updated.
-
Detection of Airborne Influenza A and SARS-CoV-2 Virus Shedding following Ocular Inoculation of Ferrets.J Virol. 2022 Dec 21;96(24):e0140322. doi: 10.1128/jvi.01403-22. Epub 2022 Nov 30. J Virol. 2022. PMID: 36448801 Free PMC article.
-
SARS-CoV-2 shedding dynamics across the respiratory tract, sex, and disease severity for adult and pediatric COVID-19.Elife. 2021 Aug 20;10:e70458. doi: 10.7554/eLife.70458. Elife. 2021. PMID: 34414888 Free PMC article.
-
Physical interventions to interrupt or reduce the spread of respiratory viruses.Cochrane Database Syst Rev. 2023 Jan 30;1(1):CD006207. doi: 10.1002/14651858.CD006207.pub6. Cochrane Database Syst Rev. 2023. PMID: 36715243 Free PMC article. Review.
-
The unique features of SARS-CoV-2 transmission: Comparison with SARS-CoV, MERS-CoV and 2009 H1N1 pandemic influenza virus.Rev Med Virol. 2021 Mar;31(2):e2171. doi: 10.1002/rmv.2171. Epub 2020 Sep 18. Rev Med Virol. 2021. PMID: 33350025 Free PMC article. Review.
Cited by
-
Superspreading of SARS-CoV-2: a systematic review and meta-analysis of event attack rates and individual transmission patterns.Epidemiol Infect. 2024 Oct 8;152:e121. doi: 10.1017/S0950268824000955. Epidemiol Infect. 2024. PMID: 39377138 Free PMC article.
-
SARS-CoV-2 aerosol transmission and detection.Eco Environ Health. 2022 Apr 15;1(1):3-10. doi: 10.1016/j.eehl.2022.03.001. eCollection 2022 Mar. Eco Environ Health. 2022. PMID: 38078196 Free PMC article. Review.
-
Determining the optimal frequency of SARS-CoV-2 regular asymptomatic testing: A randomized feasibility trial in a home care setting.PLoS One. 2024 Jul 3;19(7):e0303344. doi: 10.1371/journal.pone.0303344. eCollection 2024. PLoS One. 2024. PMID: 38959206 Free PMC article. Clinical Trial.
-
SARS-CoV-2 testing to assure safety in air travel.J Travel Med. 2021 Feb 23;28(2):taaa241. doi: 10.1093/jtm/taaa241. J Travel Med. 2021. PMID: 33410482 Free PMC article.
-
What were the historical reasons for the resistance to recognizing airborne transmission during the COVID-19 pandemic?Indoor Air. 2022 Aug;32(8):e13070. doi: 10.1111/ina.13070. Indoor Air. 2022. PMID: 36040283 Free PMC article. Review.
References
-
- Argyropoulos KV, Serrano A, Hu J, Black M, Feng X, Shen G, Call M, Kim MJ, Lytle A, Belovarac B, Vougiouklakis T, Lin LH, Moran U, Heguy A, Troxel A, Snuderl M, Osman I, Cotzia P, Jour G. Association of initial viral load in severe acute respiratory syndrome coronavirus 2 (SARS-CoV-2) Patients with outcome and symptoms. The American Journal of Pathology. 2020;190:1881–1887. doi: 10.1016/j.ajpath.2020.07.001. - DOI - PMC - PubMed
-
- Arons MM, Hatfield KM, Reddy SC, Kimball A, James A, Jacobs JR, Taylor J, Spicer K, Bardossy AC, Oakley LP, Tanwar S, Dyal JW, Harney J, Chisty Z, Bell JM, Methner M, Paul P, Carlson CM, McLaughlin HP, Thornburg N, Tong S, Tamin A, Tao Y, Uehara A, Harcourt J, Clark S, Brostrom-Smith C, Page LC, Kay M, Lewis J, Montgomery P, Stone ND, Clark TA, Honein MA, Duchin JS, Jernigan JA. Presymptomatic SARS-CoV-2 infections and transmission in a skilled nursing facility. New England Journal of Medicine. 2020;382:2081–2090. doi: 10.1056/NEJMoa2008457. - DOI - PMC - PubMed
Publication types
MeSH terms
Substances
Grants and funding
- Vanier Scholarship,608544/Natural Sciences and Engineering Research Council of Canada
- Canadian COVID-19 Rapid Research Fund,OV4-170360/CIHR/Canada
- Senior Industrial Research Chair/Natural Sciences and Engineering Research Council of Canada
- Grant/Toronto COVID-19 Action Fund
- Vanier Scholarship 608544/Natural Sciences and Engineering Research Council of Canada
LinkOut - more resources
Full Text Sources
Other Literature Sources
Medical
Miscellaneous