Mechanism of REST/NRSF regulation of clustered protocadherin α genes
- PMID: 33849071
- PMCID: PMC8096226
- DOI: 10.1093/nar/gkab248
Mechanism of REST/NRSF regulation of clustered protocadherin α genes
Abstract
Repressor element-1 silencing transcription factor (REST) or neuron-restrictive silencer factor (NRSF) is a zinc-finger (ZF) containing transcriptional repressor that recognizes thousands of neuron-restrictive silencer elements (NRSEs) in mammalian genomes. How REST/NRSF regulates gene expression remains incompletely understood. Here, we investigate the binding pattern and regulation mechanism of REST/NRSF in the clustered protocadherin (PCDH) genes. We find that REST/NRSF directionally forms base-specific interactions with NRSEs via tandem ZFs in an anti-parallel manner but with striking conformational changes. In addition, REST/NRSF recruitment to the HS5-1 enhancer leads to the decrease of long-range enhancer-promoter interactions and downregulation of the clustered PCDHα genes. Thus, REST/NRSF represses PCDHα gene expression through directional binding to a repertoire of NRSEs within the distal enhancer and variable target genes.
© The Author(s) 2021. Published by Oxford University Press on behalf of Nucleic Acids Research.
Figures
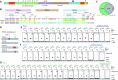
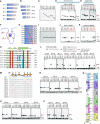
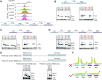
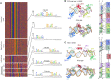
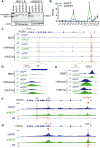
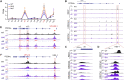
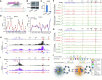
Similar articles
-
Regulatory elements required for the activation and repression of the protocadherin-alpha gene cluster.Proc Natl Acad Sci U S A. 2011 Oct 11;108(41):17195-200. doi: 10.1073/pnas.1114357108. Epub 2011 Sep 26. Proc Natl Acad Sci U S A. 2011. PMID: 21949399 Free PMC article.
-
Genome-wide identification of target genes repressed by the zinc finger transcription factor REST/NRSF in the HEK 293 cell line.Acta Biochim Biophys Sin (Shanghai). 2009 Dec;41(12):1008-17. doi: 10.1093/abbs/gmp095. Acta Biochim Biophys Sin (Shanghai). 2009. PMID: 20011975
-
Genome-wide analysis of repressor element 1 silencing transcription factor/neuron-restrictive silencing factor (REST/NRSF) target genes.Proc Natl Acad Sci U S A. 2004 Jul 13;101(28):10458-63. doi: 10.1073/pnas.0401827101. Epub 2004 Jul 6. Proc Natl Acad Sci U S A. 2004. PMID: 15240883 Free PMC article.
-
Regulation of the cholinergic gene locus by the repressor element-1 silencing transcription factor/neuron restrictive silencer factor (REST/NRSF).Life Sci. 2004 Mar 19;74(18):2213-25. doi: 10.1016/j.lfs.2003.08.045. Life Sci. 2004. PMID: 15017977 Review.
-
Brain REST/NRSF Is Not Only a Silent Repressor but Also an Active Protector.Mol Neurobiol. 2017 Jan;54(1):541-550. doi: 10.1007/s12035-015-9658-4. Epub 2016 Jan 7. Mol Neurobiol. 2017. PMID: 26742529 Review.
Cited by
-
Role of primary aging hallmarks in Alzheimer´s disease.Theranostics. 2023 Jan 1;13(1):197-230. doi: 10.7150/thno.79535. eCollection 2023. Theranostics. 2023. PMID: 36593969 Free PMC article. Review.
-
FAK-LINC01089 negative regulatory loop controls chemoresistance and progression of small cell lung cancer.Oncogene. 2024 May;43(22):1669-1687. doi: 10.1038/s41388-024-03027-y. Epub 2024 Apr 9. Oncogene. 2024. PMID: 38594505
-
Whole-genome functional characterization of RE1 silencers using a modified massively parallel reporter assay.Cell Genom. 2022 Dec 16;3(1):100234. doi: 10.1016/j.xgen.2022.100234. eCollection 2023 Jan 11. Cell Genom. 2022. PMID: 36777181 Free PMC article.
-
Systematic functional characterization of antisense eRNA of protocadherin α composite enhancer.Genes Dev. 2021 Oct 1;35(19-20):1383-1394. doi: 10.1101/gad.348621.121. Epub 2021 Sep 16. Genes Dev. 2021. PMID: 34531317 Free PMC article.
-
Investigating the Barrier Activity of Novel, Human Enhancer-Blocking Chromatin Insulators for Hematopoietic Stem Cell Gene Therapy.Hum Gene Ther. 2021 Oct;32(19-20):1186-1199. doi: 10.1089/hum.2021.142. Hum Gene Ther. 2021. PMID: 34477013 Free PMC article.
References
-
- Hsieh J., Gage F.H.. Chromatin remodeling in neural development and plasticity. Curr. Opin. Cell Biol. 2005; 17:664–671. - PubMed
-
- Chong J.A., Tapia-Ramirez J., Kim S., Toledo-Aral J.J., Zheng Y., Boutros M.C., Altshuller Y.M., Frohman M.A., Kraner S.D., Mandel G.. REST: a mammalian silencer protein that restricts sodium channel gene expression to neurons. Cell. 1995; 80:949–957. - PubMed
-
- Schoenherr C.J., Anderson D.J.. The neuron-restrictive silencer factor (NRSF): a coordinate repressor of multiple neuron-specific genes. Science. 1995; 267:1360–1363. - PubMed
-
- Ooi L., Wood I.C.. Chromatin crosstalk in development and disease: lessons from REST. Nat. Rev. Genet. 2007; 8:544–554. - PubMed
-
- Chen Z.F., Paquette A.J., Anderson D.J.. NRSF/REST is required in vivo for repression of multiple neuronal target genes during embryogenesis. Nat. Genet. 1998; 20:136–142. - PubMed
Publication types
MeSH terms
Substances
LinkOut - more resources
Full Text Sources
Other Literature Sources
Molecular Biology Databases