Engineering precision therapies: lessons and motivations from the clinic
- PMID: 33817342
- PMCID: PMC7998714
- DOI: 10.1093/synbio/ysaa024
Engineering precision therapies: lessons and motivations from the clinic
Abstract
In the past decade, gene- and cell-based therapies have been at the forefront of the biomedical revolution. Synthetic biology, the engineering discipline of building sophisticated 'genetic software' to enable precise regulation of gene activities in living cells, has been a decisive success factor of these new therapies. Here, we discuss the core technologies and treatment strategies that have already gained approval for therapeutic applications in humans. We also review promising preclinical work that could either enhance the efficacy of existing treatment strategies or pave the way for new precision medicines to treat currently intractable human conditions.
Keywords: ATMP; cell-based therapies; gene therapy; synthetic biology; translational medicine.
© The Author(s) 2020. Published by Oxford University Press.
Figures
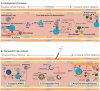
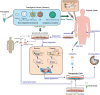
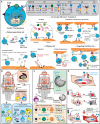
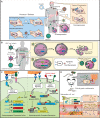
Similar articles
-
The Role of Protein Engineering in Biomedical Applications of Mammalian Synthetic Biology.Small. 2020 Jul;16(27):e1903093. doi: 10.1002/smll.201903093. Epub 2019 Oct 7. Small. 2020. PMID: 31588687 Review.
-
Engineering natural molecule-triggered genetic control systems for tunable gene- and cell-based therapies.Synth Syst Biotechnol. 2023 Jun 12;8(3):416-426. doi: 10.1016/j.synbio.2023.06.002. eCollection 2023 Sep. Synth Syst Biotechnol. 2023. PMID: 37384125 Free PMC article.
-
Designing cell function: assembly of synthetic gene circuits for cell biology applications.Nat Rev Mol Cell Biol. 2018 Aug;19(8):507-525. doi: 10.1038/s41580-018-0024-z. Nat Rev Mol Cell Biol. 2018. PMID: 29858606 Review.
-
Advancing precision medicines for ocular disorders: Diagnostic genomics to tailored therapies.Front Med (Lausanne). 2022 Jul 15;9:906482. doi: 10.3389/fmed.2022.906482. eCollection 2022. Front Med (Lausanne). 2022. PMID: 35911417 Free PMC article. Review.
-
Synthetic Biology--Toward Therapeutic Solutions.J Mol Biol. 2016 Feb 27;428(5 Pt B):945-62. doi: 10.1016/j.jmb.2015.08.020. Epub 2015 Sep 1. J Mol Biol. 2016. PMID: 26334368 Review.
Cited by
-
Synthetic Biology: Emerging Concepts to Design and Advance Adeno-Associated Viral Vectors for Gene Therapy.Adv Sci (Weinh). 2021 Feb 26;8(9):2004018. doi: 10.1002/advs.202004018. eCollection 2021 May. Adv Sci (Weinh). 2021. PMID: 33977059 Free PMC article. Review.
-
AAV-delivered muscone-induced transgene system for treating chronic diseases in mice via inhalation.Nat Commun. 2024 Feb 6;15(1):1122. doi: 10.1038/s41467-024-45383-z. Nat Commun. 2024. PMID: 38321056 Free PMC article.
-
GMP-Grade Manufacturing and Quality Control of a Non-Virally Engineered Advanced Therapy Medicinal Product for Personalized Treatment of Age-Related Macular Degeneration.Biomedicines. 2022 Nov 1;10(11):2777. doi: 10.3390/biomedicines10112777. Biomedicines. 2022. PMID: 36359296 Free PMC article.
References
-
- Bailey S.R., Maus M.V. (2019) Gene editing for immune cell therapies. Nat. Biotechnol., 37, 1425–1434. - PubMed
-
- Ma C.C., Wang Z.L., Xu T., He Z.Y., Wei Y.Q. (2019) The approved gene therapy drugs worldwide: from 1998 to 2019. Biotechnol. Adv., 107502. - PubMed
-
- Naldini L. (2015) Gene therapy returns to centre stage. Nature, 526, 351–360. - PubMed
Publication types
LinkOut - more resources
Full Text Sources
Miscellaneous