Integration of Data from Liquid-Liquid Phase Separation Databases Highlights Concentration and Dosage Sensitivity of LLPS Drivers
- PMID: 33809541
- PMCID: PMC8002189
- DOI: 10.3390/ijms22063017
Integration of Data from Liquid-Liquid Phase Separation Databases Highlights Concentration and Dosage Sensitivity of LLPS Drivers
Abstract
Liquid-liquid phase separation (LLPS) is a molecular process that leads to the formation of membraneless organelles, representing functionally specialized liquid-like cellular condensates formed by proteins and nucleic acids. Integrating the data on LLPS-associated proteins from dedicated databases revealed only modest agreement between them and yielded a high-confidence dataset of 89 human LLPS drivers. Analysis of the supporting evidence for our dataset uncovered a systematic and potentially concerning difference between protein concentrations used in a good fraction of the in vitro LLPS experiments, a key parameter that governs the phase behavior, and the proteomics-derived cellular abundance levels of the corresponding proteins. Closer scrutiny of the underlying experimental data enabled us to offer a sound rationale for this systematic difference, which draws on our current understanding of the cellular organization of the proteome and the LLPS process. In support of this rationale, we find that genes coding for our human LLPS drivers tend to be dosage-sensitive, suggesting that their cellular availability is tightly regulated to preserve their functional role in direct or indirect relation to condensate formation. Our analysis offers guideposts for increasing agreement between in vitro and in vivo studies, probing the roles of proteins in LLPS.
Keywords: data integration; dosage sensitivity; liquid demixing; liquid–liquid phase separation; local concentration; membraneless organelles; protein abundance; quantitative proteomics.
Conflict of interest statement
The authors declare no conflict of interest.
Figures
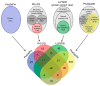
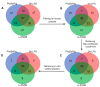
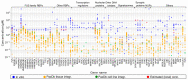
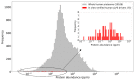
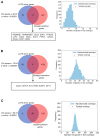
Similar articles
-
Computational resources for identifying and describing proteins driving liquid-liquid phase separation.Brief Bioinform. 2021 Sep 2;22(5):bbaa408. doi: 10.1093/bib/bbaa408. Brief Bioinform. 2021. PMID: 33517364 Free PMC article.
-
DrLLPS: a data resource of liquid-liquid phase separation in eukaryotes.Nucleic Acids Res. 2020 Jan 8;48(D1):D288-D295. doi: 10.1093/nar/gkz1027. Nucleic Acids Res. 2020. PMID: 31691822 Free PMC article.
-
Phase Separation of Epstein-Barr Virus EBNA2 and Its Coactivator EBNALP Controls Gene Expression.J Virol. 2020 Mar 17;94(7):e01771-19. doi: 10.1128/JVI.01771-19. Print 2020 Mar 17. J Virol. 2020. PMID: 31941785 Free PMC article.
-
Liquid-liquid phase separation (LLPS) in cellular physiology and tumor biology.Am J Cancer Res. 2021 Aug 15;11(8):3766-3776. eCollection 2021. Am J Cancer Res. 2021. PMID: 34522448 Free PMC article. Review.
-
Liquid-Liquid Phase Separation in Crowded Environments.Int J Mol Sci. 2020 Aug 17;21(16):5908. doi: 10.3390/ijms21165908. Int J Mol Sci. 2020. PMID: 32824618 Free PMC article. Review.
Cited by
-
Comparison of Biomolecular Condensate Localization and Protein Phase Separation Predictors.Biomolecules. 2023 Mar 13;13(3):527. doi: 10.3390/biom13030527. Biomolecules. 2023. PMID: 36979462 Free PMC article.
-
Seeing Keratinocyte Proteins through the Looking Glass of Intrinsic Disorder.Int J Mol Sci. 2021 Jul 24;22(15):7912. doi: 10.3390/ijms22157912. Int J Mol Sci. 2021. PMID: 34360678 Free PMC article. Review.
-
Novel Covalent Modifier-Induced Local Conformational Changes within the Intrinsically Disordered Region of the Androgen Receptor.Biology (Basel). 2023 Nov 17;12(11):1442. doi: 10.3390/biology12111442. Biology (Basel). 2023. PMID: 37998041 Free PMC article.
-
Transcription Regulators and Membraneless Organelles Challenges to Investigate Them.Int J Mol Sci. 2021 Nov 25;22(23):12758. doi: 10.3390/ijms222312758. Int J Mol Sci. 2021. PMID: 34884563 Free PMC article. Review.
-
Modeling Concentration-dependent Phase Separation Processes Involving Peptides and RNA via Residue-Based Coarse-Graining.J Chem Theory Comput. 2023 Jan 6:10.1021/acs.jctc.2c00856. doi: 10.1021/acs.jctc.2c00856. Online ahead of print. J Chem Theory Comput. 2023. PMID: 36607820 Free PMC article.
References
MeSH terms
Substances
Grants and funding
LinkOut - more resources
Full Text Sources
Other Literature Sources
Medical