Perinatal Hypoxemia and Oxygen Sensing
- PMID: 33792908
- PMCID: PMC8163069
- DOI: 10.1002/cphy.c190046
Perinatal Hypoxemia and Oxygen Sensing
Abstract
The development of the control of breathing begins in utero and continues postnatally. Fetal breathing movements are needed for establishing connectivity between the lungs and central mechanisms controlling breathing. Maturation of the control of breathing, including the increase of hypoxia chemosensitivity, continues postnatally. Insufficient oxygenation, or hypoxia, is a major stressor that can manifest for different reasons in the fetus and neonate. Though the fetus and neonate have different hypoxia sensing mechanisms and respond differently to acute hypoxia, both responses prevent deviations to respiratory and other developmental processes. Intermittent and chronic hypoxia pose much greater threats to the normal developmental respiratory processes. Gestational intermittent hypoxia, due to maternal sleep-disordered breathing and sleep apnea, increases eupneic breathing and decreases the hypoxic ventilatory response associated with impaired gasping and autoresuscitation postnatally. Chronic fetal hypoxia, due to biologic or environmental (i.e. high-altitude) factors, is implicated in fetal growth restriction and preterm birth causing a decrease in the postnatal hypoxic ventilatory responses with increases in irregular eupneic breathing. Mechanisms driving these changes include delayed chemoreceptor development, catecholaminergic activity, abnormal myelination, increased astrocyte proliferation in the dorsal respiratory group, among others. Long-term high-altitude residents demonstrate favorable adaptations to chronic hypoxia as do their offspring. Neonatal intermittent hypoxia is common among preterm infants due to immature respiratory systems and thus, display a reduced drive to breathe and apneas due to insufficient hypoxic sensitivity. However, ongoing intermittent hypoxia can enhance hypoxic sensitivity causing ventilatory overshoots followed by apnea; the number of apneas is positively correlated with degree of hypoxic sensitivity in preterm infants. Chronic neonatal hypoxia may arise from fetal complications like maternal smoking or from postnatal cardiovascular problems, causing blunting of the hypoxic ventilatory responses throughout at least adolescence due to attenuation of carotid body fibers responses to hypoxia with potential roles of brainstem serotonin, microglia, and inflammation, though these effects depend on the age in which chronic hypoxia initiates. Fetal and neonatal intermittent and chronic hypoxia are implicated in preterm birth and complicate the respiratory system through their direct effects on hypoxia sensing mechanisms and interruptions to the normal developmental processes. Thus, precise regulation of oxygen homeostasis is crucial for normal development of the respiratory control network. © 2021 American Physiological Society. Compr Physiol 11:1653-1677, 2021.
Copyright © 2021 American Physiological Society. All rights reserved.
Figures
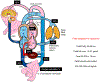
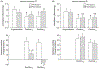
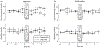
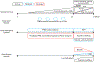
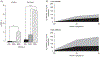
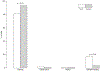
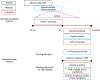
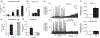
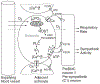
Similar articles
-
Maturation of cardioventilatory physiological trajectories in extremely preterm infants.Pediatr Res. 2024 Mar;95(4):1060-1069. doi: 10.1038/s41390-023-02839-0. Epub 2023 Oct 19. Pediatr Res. 2024. PMID: 37857848 Free PMC article.
-
Ventilatory changes during intermittent hypoxia: importance of pattern and duration.High Alt Med Biol. 2002 Summer;3(2):195-204. doi: 10.1089/15270290260131920. High Alt Med Biol. 2002. PMID: 12162863 Review.
-
Respiratory and cardiovascular responses to hypoxemia and the effects of anesthesia.Int Anesthesiol Clin. 1981 Fall;19(3):85-122. doi: 10.1097/00004311-198119030-00008. Int Anesthesiol Clin. 1981. PMID: 7026455 Review.
-
Assessing ventilatory control in infants at high risk of sleep disordered breathing: a study of infants with cleft lip and/or palate.Pediatr Pulmonol. 2013 Mar;48(3):265-73. doi: 10.1002/ppul.22568. Epub 2012 Apr 23. Pediatr Pulmonol. 2013. PMID: 22528960
-
Mechanisms of maladaptive responses of peripheral chemoreceptors to intermittent hypoxia in sleep-disordered breathing.Sheng Li Xue Bao. 2014 Feb 25;66(1):23-9. Sheng Li Xue Bao. 2014. PMID: 24553866 Review.
Cited by
-
Respiratory responses to hypoxia during rest and exercise in individuals born pre-term: a state-of-the-art review.Eur J Appl Physiol. 2022 Sep;122(9):1991-2003. doi: 10.1007/s00421-022-04965-9. Epub 2022 May 19. Eur J Appl Physiol. 2022. PMID: 35589858 Review.
-
The Oxygen Cascade from Atmosphere to Mitochondria as a Tool to Understand the (Mal)adaptation to Hypoxia.Int J Mol Sci. 2023 Feb 12;24(4):3670. doi: 10.3390/ijms24043670. Int J Mol Sci. 2023. PMID: 36835089 Free PMC article. Review.
-
Oxygen and mechanical stretch in the developing lung: risk factors for neonatal and pediatric lung disease.Front Med (Lausanne). 2023 Jun 19;10:1214108. doi: 10.3389/fmed.2023.1214108. eCollection 2023. Front Med (Lausanne). 2023. PMID: 37404808 Free PMC article. Review.
References
-
- Abu-Shaweesh JM, Martin RJ. Neonatal apnea: What’s new? Pediatr Pulmonol 43 (10): 937–944, 2008. - PubMed
-
- Adzick NS, Harrison MR, Glick PL, Villa RL, Finkbeiner W. Experimental pulmonary hypoplasia and oligohydramnios: Relative contributions of lung fluid and fetal breathing movements. J Pediatr Surg 19(6): 658–665, 1984. - PubMed
-
- Al-Matary A, Kutbi I, Qurashi M, Khalil M, Alvaro R, Kwiatkowski K, Cates D, Rigatto H. Increased peripheral chemoreceptor activity may be critical in destabilizing breathing in neonates. Semin Perinatol 28 (4): 264–272, 2004. - PubMed
-
- Alvaro R, Rigatto H. Control of breathing in newborns. In: Bracci R, Buonocore G, Weindling M, editors. Neonatology. Milano: Springer, 2012.
MeSH terms
Substances
Grants and funding
LinkOut - more resources
Full Text Sources
Other Literature Sources
Medical