The antigenic anatomy of SARS-CoV-2 receptor binding domain
- PMID: 33756110
- PMCID: PMC7891125
- DOI: 10.1016/j.cell.2021.02.032
The antigenic anatomy of SARS-CoV-2 receptor binding domain
Abstract
Antibodies are crucial to immune protection against SARS-CoV-2, with some in emergency use as therapeutics. Here, we identify 377 human monoclonal antibodies (mAbs) recognizing the virus spike and focus mainly on 80 that bind the receptor binding domain (RBD). We devise a competition data-driven method to map RBD binding sites. We find that although antibody binding sites are widely dispersed, neutralizing antibody binding is focused, with nearly all highly inhibitory mAbs (IC50 < 0.1 μg/mL) blocking receptor interaction, except for one that binds a unique epitope in the N-terminal domain. Many of these neutralizing mAbs use public V-genes and are close to germline. We dissect the structural basis of recognition for this large panel of antibodies through X-ray crystallography and cryoelectron microscopy of 19 Fab-antigen structures. We find novel binding modes for some potently inhibitory antibodies and demonstrate that strongly neutralizing mAbs protect, prophylactically or therapeutically, in animal models.
Keywords: coronavirus, SARS-CoV-2, anti-RBD antibody, receptor binding domain, antibody, immune responses, virus structure.
Copyright © 2021 The Authors. Published by Elsevier Inc. All rights reserved.
Conflict of interest statement
Declaration of interests M.S.D. is a consultant for Inbios, Vir Biotechnology, NGM Biopharmaceuticals, and Carnival Corporation and is on the Scientific Advisory Boards of Moderna and Immunome. The M.S.D. laboratory has received unrelated funding support in sponsored research agreements from Moderna, Vir Biotechnology, and Emergent BioSolutions. G.R.S. sits on the GSK Vaccines Scientific Advisory Board. A.J.P. is Chair of UK Department Health and Social Care’s (DHSC) Joint Committee on Vaccination & Immunisation (JCVI), but does not chair or participate in the JCVI COVID19 committee, and is a member of the WHO’s SAGE. The views expressed in this article do not necessarily represent the views of DHSC, JCVI, NIHR, or WHO. The University of Oxford has entered into a partnership with AstraZeneca on coronavirus vaccine development. All other authors declare no competing financial interests. The University of Oxford has protected intellectual property disclosed in this publication.
Figures
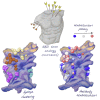
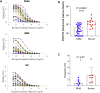
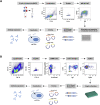
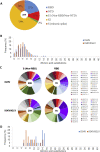
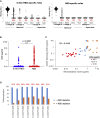
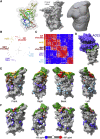
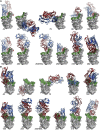
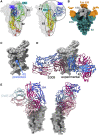
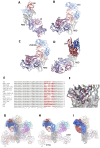
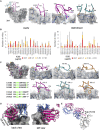
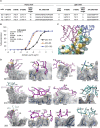
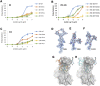
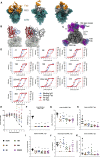
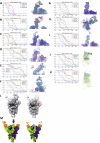
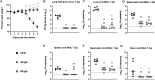
Similar articles
-
Structural Basis of a Human Neutralizing Antibody Specific to the SARS-CoV-2 Spike Protein Receptor-Binding Domain.Microbiol Spectr. 2021 Oct 31;9(2):e0135221. doi: 10.1128/Spectrum.01352-21. Epub 2021 Oct 13. Microbiol Spectr. 2021. PMID: 34643438 Free PMC article.
-
Isolation and Characterization of Mouse Monoclonal Antibodies That Neutralize SARS-CoV-2 and Its Variants of Concern Alpha, Beta, Gamma and Delta by Binding Conformational Epitopes of Glycosylated RBD With High Potency.Front Immunol. 2021 Oct 26;12:750386. doi: 10.3389/fimmu.2021.750386. eCollection 2021. Front Immunol. 2021. PMID: 34764961 Free PMC article.
-
The antibody response to SARS-CoV-2 Beta underscores the antigenic distance to other variants.Cell Host Microbe. 2022 Jan 12;30(1):53-68.e12. doi: 10.1016/j.chom.2021.11.013. Epub 2021 Nov 27. Cell Host Microbe. 2022. PMID: 34921776 Free PMC article.
-
Targeting SARS-CoV2 Spike Protein Receptor Binding Domain by Therapeutic Antibodies.Biomed Pharmacother. 2020 Oct;130:110559. doi: 10.1016/j.biopha.2020.110559. Epub 2020 Aug 1. Biomed Pharmacother. 2020. PMID: 32768882 Free PMC article. Review.
-
Structural Analysis of Neutralizing Epitopes of the SARS-CoV-2 Spike to Guide Therapy and Vaccine Design Strategies.Viruses. 2021 Jan 19;13(1):134. doi: 10.3390/v13010134. Viruses. 2021. PMID: 33477902 Free PMC article. Review.
Cited by
-
Unveiling the Interplay-Vitamin D and ACE-2 Molecular Interactions in Mitigating Complications and Deaths from SARS-CoV-2.Biology (Basel). 2024 Oct 16;13(10):831. doi: 10.3390/biology13100831. Biology (Basel). 2024. PMID: 39452140 Free PMC article. Review.
-
Characterisation of the antibody-mediated selective pressure driving intra-host evolution of SARS-CoV-2 in prolonged infection.PLoS Pathog. 2024 Oct 15;20(10):e1012624. doi: 10.1371/journal.ppat.1012624. eCollection 2024 Oct. PLoS Pathog. 2024. PMID: 39405332 Free PMC article.
-
Comparison of the Neutralization Power of Sotrovimab Against SARS-CoV-2 Variants: Development of a Rapid Computational Method.JMIR Bioinform Biotechnol. 2024 Oct 10;5:e58018. doi: 10.2196/58018. JMIR Bioinform Biotechnol. 2024. PMID: 39388246 Free PMC article.
-
Oligomerization-driven avidity correlates with SARS-CoV-2 cellular binding and inhibition.Proc Natl Acad Sci U S A. 2024 Oct;121(40):e2403260121. doi: 10.1073/pnas.2403260121. Epub 2024 Sep 19. Proc Natl Acad Sci U S A. 2024. PMID: 39298475 Free PMC article.
-
The S2 subunit of spike encodes diverse targets for functional antibody responses to SARS-CoV-2.PLoS Pathog. 2024 Aug 2;20(8):e1012383. doi: 10.1371/journal.ppat.1012383. eCollection 2024 Aug. PLoS Pathog. 2024. PMID: 39093891 Free PMC article.
References
-
- Aricescu A.R., Lu W., Jones E.Y. A time- and cost-efficient system for high-level protein production in mammalian cells. Acta Crystallogr Sect D Biol Crystallogr. 2006;62:1243–1250. - PubMed
-
- Barnes C.O., West A.P., Jr., Huey-Tubman K.E., Hoffmann M.A.G., Sharaf N.G., Hoffman P.R., Koranda N., Gristick H.B., Gaebler C., Muecksch F. Structures of Human Antibodies Bound to SARS-CoV-2 Spike Reveal Common Epitopes and Recurrent Features of Antibodies. Cell. 2020;182:828–842.e16. - PMC - PubMed
Publication types
MeSH terms
Substances
Grants and funding
- 203224/Z/16/Z/WT_/Wellcome Trust/United Kingdom
- R01 AI157155/AI/NIAID NIH HHS/United States
- MR/N00065X/1/MRC_/Medical Research Council/United Kingdom
- MR/V001329/1/MRC_/Medical Research Council/United Kingdom
- T32 AI007172/AI/NIAID NIH HHS/United States
- MC_PC_19060/MRC_/Medical Research Council/United Kingdom
- WT_/Wellcome Trust/United Kingdom
- MC_PC_20016/MRC_/Medical Research Council/United Kingdom
- 109965/Z/15/Z/WT_/Wellcome Trust/United Kingdom
- MC_UU_00008/11/MRC_/Medical Research Council/United Kingdom
- MC_PC_17137/MRC_/Medical Research Council/United Kingdom
- MR/L018942/1/MRC_/Medical Research Council/United Kingdom
- G0600520/MRC_/Medical Research Council/United Kingdom
- G1001046/MRC_/Medical Research Council/United Kingdom
LinkOut - more resources
Full Text Sources
Other Literature Sources
Medical
Molecular Biology Databases
Miscellaneous