The evolutionary history of ACE2 usage within the coronavirus subgenus Sarbecovirus
- PMID: 33754082
- PMCID: PMC7928622
- DOI: 10.1093/ve/veab007
The evolutionary history of ACE2 usage within the coronavirus subgenus Sarbecovirus
Abstract
Severe acute respiratory syndrome coronavirus 1 (SARS-CoV-1) and SARS-CoV-2 are not phylogenetically closely related; however, both use the angiotensin-converting enzyme 2 (ACE2) receptor in humans for cell entry. This is not a universal sarbecovirus trait; for example, many known sarbecoviruses related to SARS-CoV-1 have two deletions in the receptor binding domain of the spike protein that render them incapable of using human ACE2. Here, we report three sequences of a novel sarbecovirus from Rwanda and Uganda that are phylogenetically intermediate to SARS-CoV-1 and SARS-CoV-2 and demonstrate via in vitro studies that they are also unable to utilize human ACE2. Furthermore, we show that the observed pattern of ACE2 usage among sarbecoviruses is best explained by recombination not of SARS-CoV-2, but of SARS-CoV-1 and its relatives. We show that the lineage that includes SARS-CoV-2 is most likely the ancestral ACE2-using lineage, and that recombination with at least one virus from this group conferred ACE2 usage to the lineage including SARS-CoV-1 at some time in the past. We argue that alternative scenarios such as convergent evolution are much less parsimonious; we show that biogeography and patterns of host tropism support the plausibility of a recombination scenario, and we propose a competitive release hypothesis to explain how this recombination event could have occurred and why it is evolutionarily advantageous. The findings provide important insights into the natural history of ACE2 usage for both SARS-CoV-1 and SARS-CoV-2 and a greater understanding of the evolutionary mechanisms that shape zoonotic potential of coronaviruses. This study also underscores the need for increased surveillance for sarbecoviruses in southwestern China, where most ACE2-using viruses have been found to date, as well as other regions such as Africa, where these viruses have only recently been discovered.
Keywords: coronavirus; recombination; viral ecology; virus evolution.
© The Author(s) 2021. Published by Oxford University Press.
Figures
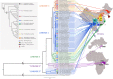
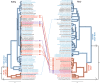
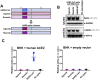
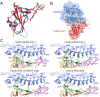
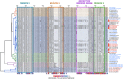
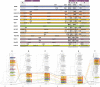
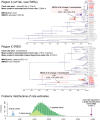
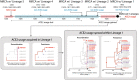
Update of
-
The evolutionary history of ACE2 usage within the coronavirus subgenus Sarbecovirus.bioRxiv [Preprint]. 2021 Jan 22:2020.07.07.190546. doi: 10.1101/2020.07.07.190546. bioRxiv. 2021. Update in: Virus Evol. 2021 Feb 05;7(1):veab007. doi: 10.1093/ve/veab007 PMID: 32676605 Free PMC article. Updated. Preprint.
Similar articles
-
The evolutionary history of ACE2 usage within the coronavirus subgenus Sarbecovirus.bioRxiv [Preprint]. 2021 Jan 22:2020.07.07.190546. doi: 10.1101/2020.07.07.190546. bioRxiv. 2021. Update in: Virus Evol. 2021 Feb 05;7(1):veab007. doi: 10.1093/ve/veab007 PMID: 32676605 Free PMC article. Updated. Preprint.
-
ACE2-Independent Bat Sarbecovirus Entry and Replication in Human and Bat Cells.mBio. 2022 Dec 20;13(6):e0256622. doi: 10.1128/mbio.02566-22. Epub 2022 Nov 21. mBio. 2022. PMID: 36409074 Free PMC article.
-
Sequence determinants of human-cell entry identified in ACE2-independent bat sarbecoviruses: A combined laboratory and computational network science approach.EBioMedicine. 2022 May;79:103990. doi: 10.1016/j.ebiom.2022.103990. Epub 2022 Apr 8. EBioMedicine. 2022. PMID: 35405384 Free PMC article.
-
[Source of the COVID-19 pandemic: ecology and genetics of coronaviruses (Betacoronavirus: Coronaviridae) SARS-CoV, SARS-CoV-2 (subgenus Sarbecovirus), and MERS-CoV (subgenus Merbecovirus).].Vopr Virusol. 2020;65(2):62-70. doi: 10.36233/0507-4088-2020-65-2-62-70. Vopr Virusol. 2020. PMID: 32515561 Review. Russian.
-
ACE2-using merbecoviruses: Further evidence of convergent evolution of ACE2 recognition by NeoCoV and other MERS-CoV related viruses.Cell Insight. 2024 Jan 30;3(1):100145. doi: 10.1016/j.cellin.2023.100145. eCollection 2024 Feb. Cell Insight. 2024. PMID: 38476250 Free PMC article. Review.
Cited by
-
Full Genome Nobecovirus Sequences From Malagasy Fruit Bats Define a Unique Evolutionary History for This Coronavirus Clade.Front Public Health. 2022 Feb 11;10:786060. doi: 10.3389/fpubh.2022.786060. eCollection 2022. Front Public Health. 2022. PMID: 35223729 Free PMC article.
-
Mutational landscape and in silico structure models of SARS-CoV-2 spike receptor binding domain reveal key molecular determinants for virus-host interaction.BMC Mol Cell Biol. 2022 Jan 7;23(1):2. doi: 10.1186/s12860-021-00403-4. BMC Mol Cell Biol. 2022. PMID: 34991443 Free PMC article.
-
Intronic regulation of SARS-CoV-2 receptor (ACE2) expression mediated by immune signaling and oxidative stress pathways.iScience. 2022 Jul 15;25(7):104614. doi: 10.1016/j.isci.2022.104614. Epub 2022 Jun 15. iScience. 2022. PMID: 35756893 Free PMC article.
-
Coronavirus and Paramyxovirus Shedding by Bats in a Cave and Buildings in Ethiopia.Ecohealth. 2022 Jun;19(2):216-232. doi: 10.1007/s10393-022-01590-y. Epub 2022 Jun 30. Ecohealth. 2022. PMID: 35771308 Free PMC article.
-
One year into the pandemic: Short-term evolution of SARS-CoV-2 and emergence of new lineages.Infect Genet Evol. 2021 Aug;92:104869. doi: 10.1016/j.meegid.2021.104869. Epub 2021 Apr 26. Infect Genet Evol. 2021. PMID: 33915216 Free PMC article. Review.
References
-
- Boni M. F. et al. (2020) ‘ Evolutionary Origins of the SARS-CoV-2 Sarbecovirus Lineage Responsible for the COVID-19 Pandemic’, Nature Microbiology, 5: 1408–17. - PubMed
Grants and funding
LinkOut - more resources
Full Text Sources
Other Literature Sources
Miscellaneous