High-Altitude Adaptation: Mechanistic Insights from Integrated Genomics and Physiology
- PMID: 33751123
- PMCID: PMC8233491
- DOI: 10.1093/molbev/msab064
High-Altitude Adaptation: Mechanistic Insights from Integrated Genomics and Physiology
Abstract
Population genomic analyses of high-altitude humans and other vertebrates have identified numerous candidate genes for hypoxia adaptation, and the physiological pathways implicated by such analyses suggest testable hypotheses about underlying mechanisms. Studies of highland natives that integrate genomic data with experimental measures of physiological performance capacities and subordinate traits are revealing associations between genotypes (e.g., hypoxia-inducible factor gene variants) and hypoxia-responsive phenotypes. The subsequent search for causal mechanisms is complicated by the fact that observed genotypic associations with hypoxia-induced phenotypes may reflect second-order consequences of selection-mediated changes in other (unmeasured) traits that are coupled with the focal trait via feedback regulation. Manipulative experiments to decipher circuits of feedback control and patterns of phenotypic integration can help identify causal relationships that underlie observed genotype-phenotype associations. Such experiments are critical for correct inferences about phenotypic targets of selection and mechanisms of adaptation.
Keywords: EPAS1; adaptation; altitude; genotype–phenotype association; hypoxia; hypoxia-inducible factor.
© The Author(s) 2021. Published by Oxford University Press on behalf of the Society for Molecular Biology and Evolution.
Figures

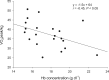
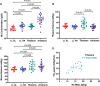
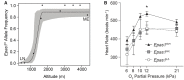
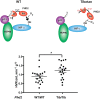
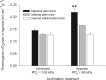
Similar articles
-
Adaptive genetic changes related to haemoglobin concentration in native high-altitude Tibetans.Exp Physiol. 2015 Nov;100(11):1263-8. doi: 10.1113/EP085035. Exp Physiol. 2015. PMID: 26454145 Review.
-
Human adaptation to the hypoxia of high altitude: the Tibetan paradigm from the pregenomic to the postgenomic era.J Appl Physiol (1985). 2014 Apr 1;116(7):875-84. doi: 10.1152/japplphysiol.00605.2013. Epub 2013 Nov 7. J Appl Physiol (1985). 2014. PMID: 24201705 Free PMC article. Review.
-
Down-Regulation of EPAS1 Transcription and Genetic Adaptation of Tibetans to High-Altitude Hypoxia.Mol Biol Evol. 2017 Apr 1;34(4):818-830. doi: 10.1093/molbev/msw280. Mol Biol Evol. 2017. PMID: 28096303 Free PMC article.
-
Physiological and genomic evidence that selection on the transcription factor Epas1 has altered cardiovascular function in high-altitude deer mice.PLoS Genet. 2019 Nov 7;15(11):e1008420. doi: 10.1371/journal.pgen.1008420. eCollection 2019 Nov. PLoS Genet. 2019. PMID: 31697676 Free PMC article.
-
Tibetans living at sea level have a hyporesponsive hypoxia-inducible factor system and blunted physiological responses to hypoxia.J Appl Physiol (1985). 2014 Apr 1;116(7):893-904. doi: 10.1152/japplphysiol.00535.2013. Epub 2013 Sep 12. J Appl Physiol (1985). 2014. PMID: 24030663 Free PMC article.
Cited by
-
Positive end-expiratory pressure and risk of postoperative pulmonary complications in patients living at high altitudes and undergoing surgery at low altitudes: a single-centre, retrospective observational study in China.BMJ Open. 2022 Jun 14;12(6):e057698. doi: 10.1136/bmjopen-2021-057698. BMJ Open. 2022. PMID: 35701068 Free PMC article.
-
Influence of High Hemoglobin-Oxygen Affinity on Humans During Hypoxia.Front Physiol. 2022 Jan 14;12:763933. doi: 10.3389/fphys.2021.763933. eCollection 2021. Front Physiol. 2022. PMID: 35095551 Free PMC article. Review.
-
Inside the Alterations of Circulating Metabolome in Antarctica: The Adaptation to Chronic Hypoxia.Front Physiol. 2022 Jan 25;13:819345. doi: 10.3389/fphys.2022.819345. eCollection 2022. Front Physiol. 2022. PMID: 35145434 Free PMC article.
-
Increased Reliance on Carbohydrates for Aerobic Exercise in Highland Andean Leaf-Eared Mice, but Not in Highland Lima Leaf-Eared Mice.Metabolites. 2021 Oct 29;11(11):750. doi: 10.3390/metabo11110750. Metabolites. 2021. PMID: 34822408 Free PMC article.
-
Deep phenotyping of 11,880 highlanders reveals novel adaptive traits in native Tibetans.iScience. 2023 Aug 18;26(9):107677. doi: 10.1016/j.isci.2023.107677. eCollection 2023 Sep 15. iScience. 2023. PMID: 37680474 Free PMC article.
References
-
- Aggarwal S, Negi S, Jha P, Singh PK, Stobdan T, Pasha MAQ, Ghosh S, Agrawal A, Prasher B, Mukerji M, et al.2010. EGLN1 involvement in high-altitude adaptation revealed through genetic analysis of extreme constitution types defined in Ayurveda. Proc Natl Acad Sci U S A. 107(44):18961–18966. - PMC - PubMed
Publication types
MeSH terms
Substances
Grants and funding
LinkOut - more resources
Full Text Sources
Other Literature Sources