Vesicle-released glutamate is necessary to maintain muscle spindle afferent excitability but not dynamic sensitivity in adult mice
- PMID: 33749829
- PMCID: PMC8169621
- DOI: 10.1113/JP281182
Vesicle-released glutamate is necessary to maintain muscle spindle afferent excitability but not dynamic sensitivity in adult mice
Abstract
Key points: Muscle spindle afferents are slowly adapting low threshold mechanoreceptors that report muscle length and movement information critical for motor control and proprioception. The rapidly adapting cation channel PIEZO2 has been identified as necessary for muscle spindle afferent stretch sensitivity, although the properties of this channel suggest that additional molecular elements are necessary for mediating the complex slowly adapting response of muscle spindle afferents. We report that glutamate increases muscle spindle afferent static sensitivity in an ex vivo mouse muscle nerve preparation, although blocking glutamate packaging into vesicles by the sole vesicular glutamate transporter, VGLUT1, either pharmacologically or by transgenic knockout of one allele of VGLUT1 decreases muscle spindle afferent static but not dynamic sensitivity. Our results confirm that vesicle-released glutamate is an important contributor to maintained muscle spindle afferent excitability and may suggest a therapeutic target for normalizing muscle spindle afferent function.
Abstract: Muscle spindle afferents are slowly adapting low threshold mechanoreceptors that have both dynamic and static sensitivity to muscle stretch. The exact mechanism by which these neurons translate muscle movement into action potentials is not well understood, although the PIEZO2 mechanically sensitive cation channel is essential for stretch sensitivity. PIEZO2 is rapidly adapting, suggesting the requirement for additional molecular elements to maintain firing during stretch. Spindle afferent sensory endings contain glutamate-filled synaptic-like vesicles that are released in a stretch- and calcium-dependent manner. Previous work has shown that glutamate can increase and a phospholipase-D coupled metabotropic glutamate antagonist can abolish firing during static stretch. Here, we test the hypothesis that vesicle-released glutamate is necessary for maintaining muscle spindle afferent excitability during static but not dynamic stretch. To test this hypothesis, we used a mouse muscle-nerve ex vivo preparation to measure identified muscle spindle afferent responses to stretch and vibration. In C57BL/6 adult mice, bath applied glutamate significantly increased the firing rate during the plateau phase of stretch but not during the dynamic phase of stretch. Blocking the packaging of glutamate into vesicles by the sole vesicular glutamate transporter, VGLUT1, either with xanthurenic acid or by using a transgenic mouse with only one copy of the VGLUT1 gene (VGLUT1+/- ), decreased muscle spindle afferent firing during sustained stretch but not during vibration. Our results suggest a model of mechanotransduction where calcium entering the PIEZO2 channel can cause the release of glutamate from synaptic-like vesicles, which then helps to maintain afferent depolarization and firing.
Keywords: glutamate; mechanotransduction; muscle afferent; muscle spindle; sensory physiology.
© 2021 The Authors. The Journal of Physiology © 2021 The Physiological Society.
Conflict of interest statement
The authors declare no competing interests.
Figures
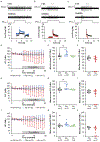
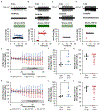
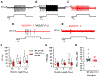
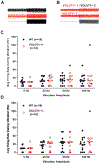
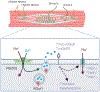
Comment in
-
Spindles are doin' it for themselves: Glutamatergic autoexcitation in muscle spindles.J Physiol. 2021 Jun;599(11):2781-2783. doi: 10.1113/JP281624. Epub 2021 May 10. J Physiol. 2021. PMID: 33871872 No abstract available.
Similar articles
-
Molecular determinants of mechanosensation in the muscle spindle.Curr Opin Neurobiol. 2022 Jun;74:102542. doi: 10.1016/j.conb.2022.102542. Epub 2022 Apr 14. Curr Opin Neurobiol. 2022. PMID: 35430481 Free PMC article. Review.
-
Acetylcholine receptors in the equatorial region of intrafusal muscle fibres modulate mouse muscle spindle sensitivity.J Physiol. 2019 Apr;597(7):1993-2006. doi: 10.1113/JP277139. Epub 2019 Feb 13. J Physiol. 2019. PMID: 30673133 Free PMC article.
-
Autogenic modulation of mechanoreceptor excitability by glutamate release from synaptic-like vesicles: evidence from the rat muscle spindle primary sensory ending.J Physiol. 2005 Jan 15;562(Pt 2):381-94. doi: 10.1113/jphysiol.2004.074799. Epub 2004 Nov 4. J Physiol. 2005. PMID: 15528245 Free PMC article.
-
Modulating mechanosensory afferent excitability by an atypical mGluR.J Anat. 2015 Aug;227(2):214-20. doi: 10.1111/joa.12319. Epub 2015 Jun 5. J Anat. 2015. PMID: 26053109 Free PMC article. Review.
-
Lipopolysaccharide-induced inflammation does not alter muscle spindle afferent mechanosensation or sensory integration in the spinal cord of adult mice.Physiol Rep. 2018 Sep;6(17):e13812. doi: 10.14814/phy2.13812. Physiol Rep. 2018. PMID: 30178608 Free PMC article.
Cited by
-
Post Orgasmic Illness Syndrome (POIS) and Delayed Onset Muscle Soreness (DOMS): Do They Have Anything in Common?Cells. 2021 Jul 23;10(8):1867. doi: 10.3390/cells10081867. Cells. 2021. PMID: 34440637 Free PMC article.
-
Psoriasis, Is It a Microdamage of Our "Sixth Sense"? A Neurocentric View.Int J Mol Sci. 2022 Oct 8;23(19):11940. doi: 10.3390/ijms231911940. Int J Mol Sci. 2022. PMID: 36233237 Free PMC article.
-
Molecular determinants of mechanosensation in the muscle spindle.Curr Opin Neurobiol. 2022 Jun;74:102542. doi: 10.1016/j.conb.2022.102542. Epub 2022 Apr 14. Curr Opin Neurobiol. 2022. PMID: 35430481 Free PMC article. Review.
-
Degeneration of muscle spindles in a murine model of Pompe disease.Sci Rep. 2023 Apr 21;13(1):6555. doi: 10.1038/s41598-023-33543-y. Sci Rep. 2023. PMID: 37085544 Free PMC article.
-
Delayed Onset Muscle Soreness and Critical Neural Microdamage-Derived Neuroinflammation.Biomolecules. 2022 Aug 31;12(9):1207. doi: 10.3390/biom12091207. Biomolecules. 2022. PMID: 36139045 Free PMC article. Review.
References
-
- Boulland JL, Qureshi T, Seal RP, Rafiki A, Gundersen V, Bergersen LH, Fremeau RT Jr, Edwards RH, Storm-Mathisen J & Chaudhry FA. (2004). Expression of the vesicular glutamate transporters during development indicates the widespread corelease of multiple neurotransmitters. Journal of Comparative Neurology 480, 264–280. - PubMed
Publication types
MeSH terms
Substances
Grants and funding
LinkOut - more resources
Full Text Sources
Other Literature Sources