Horizontal Gene Transfer and Gene Duplication of β-Fructofuranosidase Confer Lepidopteran Insects Metabolic Benefits
- PMID: 33739418
- PMCID: PMC8233494
- DOI: 10.1093/molbev/msab080
Horizontal Gene Transfer and Gene Duplication of β-Fructofuranosidase Confer Lepidopteran Insects Metabolic Benefits
Abstract
Horizontal gene transfer (HGT) is a potentially critical source of material for ecological adaptation and the evolution of novel genetic traits. However, reports on posttransfer duplication in organism genomes are lacking, and the evolutionary advantages conferred on the recipient are generally poorly understood. Sucrase plays an important role in insect physiological growth and development. Here, we performed a comprehensive analysis of the evolution of insect β-fructofuranosidase transferred from bacteria via HGT. We found that posttransfer duplications of β-fructofuranosidase were widespread in Lepidoptera and sporadic occurrences of β-fructofuranosidase were found in Coleoptera and Hymenoptera. β-fructofuranosidase genes often undergo modifications, such as gene duplication, differential gene loss, and changes in mutation rates. Lepidopteran β-fructofuranosidase gene (SUC) clusters showed marked divergence in gene expression patterns and enzymatic properties in Bombyx mori (moth) and Papilio xuthus (butterfly). We generated SUC1 mutations in B. mori using CRISPR/Cas9 to thoroughly examine the physiological function of SUC. BmSUC1 mutant larvae were viable but displayed delayed growth and reduced sucrase activities that included susceptibility to the sugar mimic alkaloid found in high concentrations in mulberry. BmSUC1 served as a critical sucrase and supported metabolic homeostasis in the larval midgut and silk gland, suggesting that gene transfer of β-fructofuranosidase enhanced the digestive and metabolic adaptation of lepidopteran insects. These findings highlight not only the universal function of β-fructofuranosidase with a link to the maintenance of carbohydrate metabolism but also an underexplored function in the silk gland. This study expands our knowledge of posttransfer duplication and subsequent functional diversification in the adaptive evolution and lineage-specific adaptation of organisms.
Keywords: β-fructofuranosidase; adaptation; gene duplication; horizontal gene transfer; insect.
© The Author(s) 2021. Published by Oxford University Press on behalf of the Society for Molecular Biology and Evolution.
Figures
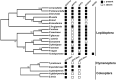
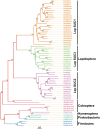
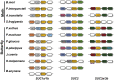
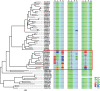
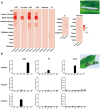
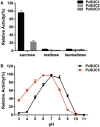
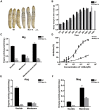
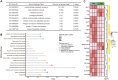
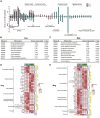
Similar articles
-
BmSuc1 Affects Silk Properties by Acting on Sericin1 in Bombyx mori.Int J Mol Sci. 2022 Aug 31;23(17):9891. doi: 10.3390/ijms23179891. Int J Mol Sci. 2022. PMID: 36077290 Free PMC article.
-
Beta-fructofuranosidase genes of the silkworm, Bombyx mori: insights into enzymatic adaptation of B. mori to toxic alkaloids in mulberry latex.J Biol Chem. 2008 May 30;283(22):15271-9. doi: 10.1074/jbc.M709350200. Epub 2008 Apr 8. J Biol Chem. 2008. PMID: 18397891 Free PMC article.
-
BmSUC1 is essential for glycometabolism modulation in the silkworm, Bombyx mori.Biochim Biophys Acta Gene Regul Mech. 2018 Jun;1861(6):543-553. doi: 10.1016/j.bbagrm.2018.04.002. Epub 2018 Apr 14. Biochim Biophys Acta Gene Regul Mech. 2018. PMID: 29660529
-
Origins of bacterial diversity through horizontal genetic transfer and adaptation to new ecological niches.FEMS Microbiol Rev. 2011 Sep;35(5):957-76. doi: 10.1111/j.1574-6976.2011.00292.x. Epub 2011 Jul 29. FEMS Microbiol Rev. 2011. PMID: 21711367 Review.
-
Gustatory receptors in Lepidoptera: chemosensation and beyond.Insect Mol Biol. 2016 Oct;25(5):519-29. doi: 10.1111/imb.12246. Epub 2016 May 26. Insect Mol Biol. 2016. PMID: 27228010 Review.
Cited by
-
Characterization and organization of telomeric-linked helicase (tlh) gene families in Fusarium oxysporum.Curr Genet. 2024 Nov 12;70(1):19. doi: 10.1007/s00294-024-01303-8. Curr Genet. 2024. PMID: 39528830
-
HGT is widespread in insects and contributes to male courtship in lepidopterans.Cell. 2022 Aug 4;185(16):2975-2987.e10. doi: 10.1016/j.cell.2022.06.014. Epub 2022 Jul 18. Cell. 2022. PMID: 35853453 Free PMC article.
-
BmSuc1 Affects Silk Properties by Acting on Sericin1 in Bombyx mori.Int J Mol Sci. 2022 Aug 31;23(17):9891. doi: 10.3390/ijms23179891. Int J Mol Sci. 2022. PMID: 36077290 Free PMC article.
References
-
- Afshar K, Dube FF, Najafabadi HS, Bonneil E, Thibault P, Salavati R, Bede JC.. 2013. Insights into the insect salivary gland proteome: diet-associated changes in caterpillar labial salivary proteins. J Insect Physiol. 59(3):351–366. - PubMed
-
- Albalat R, Cañestro C.. 2016. Evolution by gene loss. Nat Rev Genet. 17(7):379–391. - PubMed
Publication types
MeSH terms
Substances
LinkOut - more resources
Full Text Sources
Other Literature Sources