A conserved immunogenic and vulnerable site on the coronavirus spike protein delineated by cross-reactive monoclonal antibodies
- PMID: 33731724
- PMCID: PMC7969777
- DOI: 10.1038/s41467-021-21968-w
A conserved immunogenic and vulnerable site on the coronavirus spike protein delineated by cross-reactive monoclonal antibodies
Abstract
The coronavirus spike glycoprotein, located on the virion surface, is the key mediator of cell entry and the focus for development of protective antibodies and vaccines. Structural studies show exposed sites on the spike trimer that might be targeted by antibodies with cross-species specificity. Here we isolated two human monoclonal antibodies from immunized humanized mice that display a remarkable cross-reactivity against distinct spike proteins of betacoronaviruses including SARS-CoV, SARS-CoV-2, MERS-CoV and the endemic human coronavirus HCoV-OC43. Both cross-reactive antibodies target the stem helix in the spike S2 fusion subunit which, in the prefusion conformation of trimeric spike, forms a surface exposed membrane-proximal helical bundle. Both antibodies block MERS-CoV infection in cells and provide protection to mice from lethal MERS-CoV challenge in prophylactic and/or therapeutic models. Our work highlights an immunogenic and vulnerable site on the betacoronavirus spike protein enabling elicitation of antibodies with unusual binding breadth.
Conflict of interest statement
C.W., R.v.H., W.L., I.W., B.v.D., N.M.A.O., F.G., F.J.M.v.K., B.L.H., D.D., and B.J.B. are inventors on a patent application on monoclonal antibodies targeting MERS-CoV (patent publication no.: WO/2020/169755). F.G., D.D. and R.H. are non-substantial interest shareholders in Harbour Biomed and were part of the team that generated the mice. The other authors declare no competing interests.
Figures
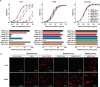
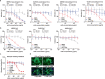
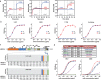
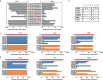
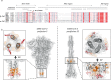
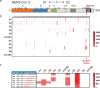
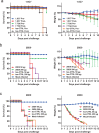
Similar articles
-
Towards a solution to MERS: protective human monoclonal antibodies targeting different domains and functions of the MERS-coronavirus spike glycoprotein.Emerg Microbes Infect. 2019;8(1):516-530. doi: 10.1080/22221751.2019.1597644. Emerg Microbes Infect. 2019. PMID: 30938227 Free PMC article.
-
Recombinant Receptor-Binding Domains of Multiple Middle East Respiratory Syndrome Coronaviruses (MERS-CoVs) Induce Cross-Neutralizing Antibodies against Divergent Human and Camel MERS-CoVs and Antibody Escape Mutants.J Virol. 2016 Dec 16;91(1):e01651-16. doi: 10.1128/JVI.01651-16. Print 2017 Jan 1. J Virol. 2016. PMID: 27795425 Free PMC article.
-
Characterization of MW06, a human monoclonal antibody with cross-neutralization activity against both SARS-CoV-2 and SARS-CoV.MAbs. 2021 Jan-Dec;13(1):1953683. doi: 10.1080/19420862.2021.1953683. MAbs. 2021. PMID: 34313527 Free PMC article.
-
Targeting SARS-CoV2 Spike Protein Receptor Binding Domain by Therapeutic Antibodies.Biomed Pharmacother. 2020 Oct;130:110559. doi: 10.1016/j.biopha.2020.110559. Epub 2020 Aug 1. Biomed Pharmacother. 2020. PMID: 32768882 Free PMC article. Review.
-
Neutralizing Monoclonal Antibodies as Promising Therapeutics against Middle East Respiratory Syndrome Coronavirus Infection.Viruses. 2018 Nov 30;10(12):680. doi: 10.3390/v10120680. Viruses. 2018. PMID: 30513619 Free PMC article. Review.
Cited by
-
Discovery and characterization of a pan-betacoronavirus S2-binding antibody.Structure. 2024 Nov 7;32(11):1893-1909.e11. doi: 10.1016/j.str.2024.08.022. Epub 2024 Sep 25. Structure. 2024. PMID: 39326419
-
Bacterial pneumonia patients with elevated globulin levels did not get infected with SARS-CoV-2: two case reports.Front Immunol. 2024 Aug 29;15:1404542. doi: 10.3389/fimmu.2024.1404542. eCollection 2024. Front Immunol. 2024. PMID: 39267743 Free PMC article.
-
Simulation-driven design of stabilized SARS-CoV-2 spike S2 immunogens.Nat Commun. 2024 Aug 27;15(1):7370. doi: 10.1038/s41467-024-50976-9. Nat Commun. 2024. PMID: 39191724 Free PMC article.
-
Dissecting humoral immune responses to an MVA-vectored MERS-CoV vaccine in humans using a systems serology approach.iScience. 2024 Jul 8;27(8):110470. doi: 10.1016/j.isci.2024.110470. eCollection 2024 Aug 16. iScience. 2024. PMID: 39148710 Free PMC article.
-
Passive infusion of an S2-Stem broadly neutralizing antibody protects against SARS-CoV-2 infection and lower airway inflammation in rhesus macaques.bioRxiv [Preprint]. 2024 Jul 30:2024.07.30.605768. doi: 10.1101/2024.07.30.605768. bioRxiv. 2024. PMID: 39109178 Free PMC article. Preprint.
References
-
- World Health Organization. MERS situation update, January 2020. http://www.emro.who.int/health-topics/mers-cov/mersoutbreaks.html (2020).
-
- Gaunt ER, Hardie A, Claas EC, Simmonds P, Templeton KE. Epidemiology and clinical presentations of the four human coronaviruses 229E, HKU1, NL63, and OC43 detected over 3 years using a novel multiplex real-time PCR method. J. Clin. Microbiol. 2010;48:2940–2947. doi: 10.1128/JCM.00636-10. - DOI - PMC - PubMed
Publication types
MeSH terms
Substances
LinkOut - more resources
Full Text Sources
Other Literature Sources
Molecular Biology Databases
Miscellaneous