Chemically Defined Xeno- and Serum-Free Cell Culture Medium to Grow Human Adipose Stem Cells
- PMID: 33671568
- PMCID: PMC7926673
- DOI: 10.3390/cells10020466
Chemically Defined Xeno- and Serum-Free Cell Culture Medium to Grow Human Adipose Stem Cells
Abstract
Adipose tissue is an abundant source of stem cells. However, liposuction cannot yield cell quantities sufficient for direct applications in regenerative medicine. Therefore, the development of GMP-compliant ex vivo expansion protocols is required to ensure the production of a "cell drug" that is safe, reproducible, and cost-effective. Thus, we developed our own basal defined xeno- and serum-free cell culture medium (UrSuppe), specifically formulated to grow human adipose stem cells (hASCs). With this medium, we can directly culture the stromal vascular fraction (SVF) cells in defined cell culture conditions to obtain hASCs. Cells proliferate while remaining undifferentiated, as shown by Flow Cytometry (FACS), Quantitative Reverse Transcription PCR (RT-qPCR) assays, and their secretion products. Using the UrSuppe cell culture medium, maximum cell densities between 0.51 and 0.80 × 105 cells/cm2 (=2.55-4.00 × 105 cells/mL) were obtained. As the expansion of hASCs represents only the first step in a cell therapeutic protocol or further basic research studies, we formulated two chemically defined media to differentiate the expanded hASCs in white or beige/brown adipocytes. These new media could help translate research projects into the clinical application of hASCs and study ex vivo the biology in healthy and dysfunctional states of adipocytes and their precursors. Following the cell culture system developers' practice and obvious reasons related to the formulas' patentability, the defined media's composition will not be disclosed in this study.
Keywords: UrSuppe; adipogenic differentiated adipose-derived stromal cells; defined cell culture; platelet lysate; white and beige/brown adipocyte; xeno- and serum-free cell culture.
Conflict of interest statement
The authors declare no conflict of interest regarding this paper’s publication.
Figures
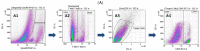
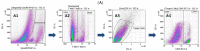
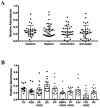
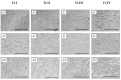
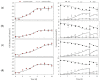
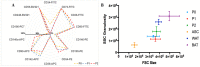
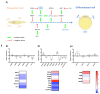
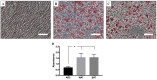
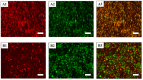
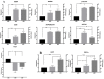
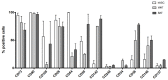
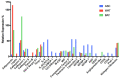
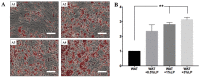
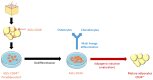
Similar articles
-
Human Adipose Stem Cells (hASCs) Grown on Biodegradable Microcarriers in Serum- and Xeno-Free Medium Preserve Their Undifferentiated Status.J Funct Biomater. 2021 Apr 16;12(2):25. doi: 10.3390/jfb12020025. J Funct Biomater. 2021. PMID: 33923488 Free PMC article.
-
Human adipose tissue-derived stem cells cultured in xeno-free culture condition enhance c-MYC expression increasing proliferation but bypassing spontaneous cell transformation.Stem Cell Res Ther. 2015 Apr 14;6(1):76. doi: 10.1186/s13287-015-0030-4. Stem Cell Res Ther. 2015. PMID: 25889298 Free PMC article.
-
Improved GMP compliant approach to manipulate lipoaspirates, to cryopreserve stromal vascular fraction, and to expand adipose stem cells in xeno-free media.Stem Cell Res Ther. 2018 May 11;9(1):130. doi: 10.1186/s13287-018-0886-1. Stem Cell Res Ther. 2018. PMID: 29751821 Free PMC article.
-
Expansion of Mesenchymal Stem/Stromal cells under xenogenic-free culture conditions.Adv Biochem Eng Biotechnol. 2013;129:33-57. doi: 10.1007/10_2012_134. Adv Biochem Eng Biotechnol. 2013. PMID: 22777242 Review.
-
Preclinical Research of Stem Cells: Challenges and Progress.Stem Cell Rev Rep. 2023 Aug;19(6):1676-1690. doi: 10.1007/s12015-023-10528-y. Epub 2023 Apr 25. Stem Cell Rev Rep. 2023. PMID: 37097496 Review.
Cited by
-
Mesenchymal and induced pluripotent stem cell-based therapeutics: a comparison.Appl Microbiol Biotechnol. 2023 Jul;107(14):4429-4445. doi: 10.1007/s00253-023-12583-4. Epub 2023 May 29. Appl Microbiol Biotechnol. 2023. PMID: 37246986 Free PMC article. Review.
-
Chemically Defined, Xeno-Free Expansion of Human Mesenchymal Stem Cells (hMSCs) on Benchtop-Scale Using a Stirred Single-Use Bioreactor.Methods Mol Biol. 2022;2436:83-111. doi: 10.1007/7651_2021_426. Methods Mol Biol. 2022. PMID: 34611815
-
Metabolic Responses and Pathway Changes of Vero Cells under High-Vitamin B Medium.Vaccines (Basel). 2022 Oct 25;10(11):1787. doi: 10.3390/vaccines10111787. Vaccines (Basel). 2022. PMID: 36366296 Free PMC article.
-
Human Adipose Stem Cells (hASCs) Grown on Biodegradable Microcarriers in Serum- and Xeno-Free Medium Preserve Their Undifferentiated Status.J Funct Biomater. 2021 Apr 16;12(2):25. doi: 10.3390/jfb12020025. J Funct Biomater. 2021. PMID: 33923488 Free PMC article.
-
Characterization of Human Subcutaneous Adipose Tissue and Validation of the Banking Procedure for Autologous Transplantation.Int J Mol Sci. 2023 May 3;24(9):8190. doi: 10.3390/ijms24098190. Int J Mol Sci. 2023. PMID: 37175896 Free PMC article.
References
Publication types
MeSH terms
LinkOut - more resources
Full Text Sources
Other Literature Sources
Medical
Research Materials