New light shed on the early evolution of limb-bone growth plate and bone marrow
- PMID: 33648627
- PMCID: PMC7924947
- DOI: 10.7554/eLife.51581
New light shed on the early evolution of limb-bone growth plate and bone marrow
Abstract
The production of blood cells (haematopoiesis) occurs in the limb bones of most tetrapods but is absent in the fin bones of ray-finned fish. When did long bones start producing blood cells? Recent hypotheses suggested that haematopoiesis migrated into long bones prior to the water-to-land transition and protected newly-produced blood cells from harsher environmental conditions. However, little fossil evidence to support these hypotheses has been provided so far. Observations of the humeral microarchitecture of stem-tetrapods, batrachians, and amniotes were performed using classical sectioning and three-dimensional synchrotron virtual histology. They show that Permian tetrapods seem to be among the first to exhibit a centralised marrow organisation, which allows haematopoiesis as in extant amniotes. Not only does our study demonstrate that long-bone haematopoiesis was probably not an exaptation to the water-to-land transition but it sheds light on the early evolution of limb-bone development and the sequence of bone-marrow functional acquisitions.
Keywords: amphibians; evolutionary biology; haematopoiesis; propagation phase-contrast synchrotron microtomography; stem amniotes; tetrapod terrestrialisation; three-dimensional virtual palaeohistology.
Plain language summary
For many aquatic creatures, the red blood cells that rush through their bodies are created in organs such as the liver or the kidney. In most land vertebrates however, blood-cell production occurs in the bone marrow. There, the process is shielded from the ultraviolet light or starker temperature changes experienced out of the water. It is possible that this difference evolved long before the first animal with a backbone crawled out of the aquatic environment and faced new, harsher conditions: yet very little fossil evidence exists to support this idea. A definitive answer demands a close examination of fossils from the water-to-land transition including lobe-finned fish and early limbed vertebrates. To support the production of red blood cells, their fin and limb bones would have needed an internal cavity that can house a specific niche that opens onto a complex network of blood vessels. To investigate this question, Estefa et al. harnessed the powerful x-ray beam produced by the European Synchrotron Radiation Facility and imaged the fin and limb bones from fossil lobe-finned fish and early limbed vertebrates. The resulting three-dimensional structures revealed spongy long bones with closed internal cavities where the bone marrow cells were probably entrapped. These could not have housed the blood vessels needed to create an environment that produces red blood cells. In fact, the earliest four-legged land animals Estefa et al. found with an open marrow cavity lived 60 million years after vertebrates had first emerged from the aquatic environment, suggesting that blood cells only began to be created in bone marrow after the water-to-land transition. Future work could help to pinpoint exactly when the change in blood cell production occurred, helping researchers to identify the environmental and biological factors that drove this change.
© 2021, Estefa et al.
Conflict of interest statement
JE, PT, AC, JK, GN, CB, SS No competing interests declared
Figures
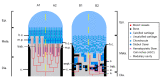
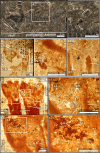
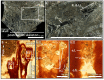
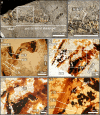
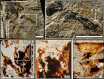
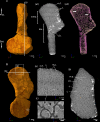
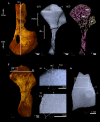
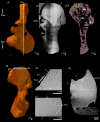
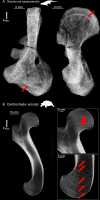
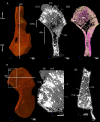
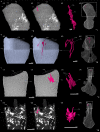
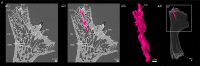
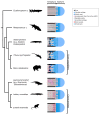
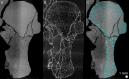
Comment in
-
Unraveling the history of limb bones.Elife. 2021 Mar 2;10:e66506. doi: 10.7554/eLife.66506. Elife. 2021. PMID: 33648629 Free PMC article.
Similar articles
-
Unraveling the history of limb bones.Elife. 2021 Mar 2;10:e66506. doi: 10.7554/eLife.66506. Elife. 2021. PMID: 33648629 Free PMC article.
-
The humerus of Eusthenopteron: a puzzling organization presaging the establishment of tetrapod limb bone marrow.Proc Biol Sci. 2014 Mar 19;281(1782):20140299. doi: 10.1098/rspb.2014.0299. Print 2014 May 7. Proc Biol Sci. 2014. PMID: 24648231 Free PMC article.
-
Reconstructing pectoral appendicular muscle anatomy in fossil fish and tetrapods over the fins-to-limbs transition.Biol Rev Camb Philos Soc. 2018 May;93(2):1077-1107. doi: 10.1111/brv.12386. Epub 2017 Nov 10. Biol Rev Camb Philos Soc. 2018. PMID: 29125205 Review.
-
Lung evolution in vertebrates and the water-to-land transition.Elife. 2022 Jul 26;11:e77156. doi: 10.7554/eLife.77156. Elife. 2022. PMID: 35880746 Free PMC article.
-
New frontiers in the evolution of fin development.J Exp Zool B Mol Dev Evol. 2014 Nov;322(7):540-52. doi: 10.1002/jez.b.22563. Epub 2014 Feb 12. J Exp Zool B Mol Dev Evol. 2014. PMID: 24677573 Review.
Cited by
-
Mineralized Cartilage and Bone-Like Tissues in Chondrichthyans Offer Potential Insights Into the Evolution and Development of Mineralized Tissues in the Vertebrate Endoskeleton.Front Genet. 2021 Dec 22;12:762042. doi: 10.3389/fgene.2021.762042. eCollection 2021. Front Genet. 2021. PMID: 35003210 Free PMC article. Review.
-
Endogenous Retroviruses Augment Amphibian (Xenopus laevis) Tadpole Antiviral Protection.J Virol. 2022 Jun 8;96(11):e0063422. doi: 10.1128/jvi.00634-22. Epub 2022 May 16. J Virol. 2022. PMID: 35575553 Free PMC article.
-
The cellular basis of cartilage growth and shape change in larval and metamorphosing Xenopus frogs.PLoS One. 2023 Jan 12;18(1):e0277110. doi: 10.1371/journal.pone.0277110. eCollection 2023. PLoS One. 2023. PMID: 36634116 Free PMC article.
-
Osteohistology of Greererpeton provides insight into the life history of an early Carboniferous tetrapod.J Anat. 2021 Dec;239(6):1256-1272. doi: 10.1111/joa.13520. Epub 2021 Jul 26. J Anat. 2021. PMID: 34310687 Free PMC article. Review.
-
Unraveling the history of limb bones.Elife. 2021 Mar 2;10:e66506. doi: 10.7554/eLife.66506. Elife. 2021. PMID: 33648629 Free PMC article.
References
-
- Ahlberg PE. Follow the footprints and mind the gaps: a new look at the origin of tetrapods. Earth and Environmental Science Transactions of the Royal Society of Edinburgh. 2018;109:115–137. doi: 10.1017/S1755691018000695. - DOI
-
- Anderson JS. Incorporating ontogeny into the matrix: a phylogenetic evaluation of developmental evidence for the origin of modern amphibians. In: Anderson J. S, Sues H. -D, editors. Major Transitions in Vertebrate Evolution. Bloomington: Indiana University Press; 2007. pp. 182–227.
Publication types
MeSH terms
Grants and funding
LinkOut - more resources
Full Text Sources
Other Literature Sources