Short antisense oligonucleotides alleviate the pleiotropic toxicity of RNA harboring expanded CGG repeats
- PMID: 33627639
- PMCID: PMC7904788
- DOI: 10.1038/s41467-021-21021-w
Short antisense oligonucleotides alleviate the pleiotropic toxicity of RNA harboring expanded CGG repeats
Abstract
Fragile X-associated tremor/ataxia syndrome (FXTAS) is an incurable neurodegenerative disorder caused by expansion of CGG repeats in the FMR1 5'UTR. The RNA containing expanded CGG repeats (rCGGexp) causes cell damage by interaction with complementary DNA, forming R-loop structures, sequestration of nuclear proteins involved in RNA metabolism and initiation of translation of polyglycine-containing protein (FMRpolyG), which forms nuclear insoluble inclusions. Here we show the therapeutic potential of short antisense oligonucleotide steric blockers (ASOs) targeting directly the rCGGexp. In nuclei of FXTAS cells ASOs affect R-loop formation and correct miRNA biogenesis and alternative splicing, indicating that nuclear proteins are released from toxic sequestration. In cytoplasm, ASOs significantly decrease the biosynthesis and accumulation of FMRpolyG. Delivery of ASO into a brain of FXTAS mouse model reduces formation of inclusions, improves motor behavior and corrects gene expression profile with marginal signs of toxicity after a few weeks from a treatment.
Conflict of interest statement
The authors declare no competing interests.
Figures
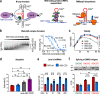
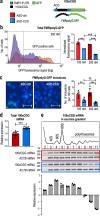
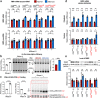
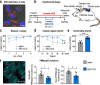
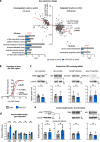
Similar articles
-
Translation of Expanded CGG Repeats into FMRpolyG Is Pathogenic and May Contribute to Fragile X Tremor Ataxia Syndrome.Neuron. 2017 Jan 18;93(2):331-347. doi: 10.1016/j.neuron.2016.12.016. Epub 2017 Jan 5. Neuron. 2017. PMID: 28065649 Free PMC article.
-
Astroglial-targeted expression of the fragile X CGG repeat premutation in mice yields RAN translation, motor deficits and possible evidence for cell-to-cell propagation of FXTAS pathology.Acta Neuropathol Commun. 2019 Feb 26;7(1):27. doi: 10.1186/s40478-019-0677-7. Acta Neuropathol Commun. 2019. PMID: 30808398 Free PMC article.
-
FMRpolyG alters mitochondrial transcripts level and respiratory chain complex assembly in Fragile X associated tremor/ataxia syndrome [FXTAS].Biochim Biophys Acta Mol Basis Dis. 2019 Jun 1;1865(6):1379-1388. doi: 10.1016/j.bbadis.2019.02.010. Epub 2019 Feb 13. Biochim Biophys Acta Mol Basis Dis. 2019. PMID: 30771487
-
Repeat-associated non-AUG (RAN) translation and other molecular mechanisms in Fragile X Tremor Ataxia Syndrome.Brain Res. 2018 Aug 15;1693(Pt A):43-54. doi: 10.1016/j.brainres.2018.02.006. Epub 2018 Feb 14. Brain Res. 2018. PMID: 29453961 Free PMC article. Review.
-
Molecular pathogenesis of fragile X-associated tremor/ataxia syndrome.J Investig Med. 2009 Dec;57(8):825-9. doi: 10.2310/JIM.0b013e3181be329a. J Investig Med. 2009. PMID: 19794313 Free PMC article. Review.
Cited by
-
RNA structure promotes liquid-to-solid phase transition of short RNAs in neuronal dysfunction.Commun Biol. 2024 Jan 29;7(1):137. doi: 10.1038/s42003-024-05828-z. Commun Biol. 2024. PMID: 38287096 Free PMC article.
-
(Dys)function Follows Form: Nucleic Acid Structure, Repeat Expansion, and Disease Pathology in FMR1 Disorders.Int J Mol Sci. 2021 Aug 25;22(17):9167. doi: 10.3390/ijms22179167. Int J Mol Sci. 2021. PMID: 34502075 Free PMC article. Review.
-
Non-canonical DNA/RNA structures associated with the pathogenesis of Fragile X-associated tremor/ataxia syndrome and Fragile X syndrome.Front Genet. 2022 Aug 30;13:866021. doi: 10.3389/fgene.2022.866021. eCollection 2022. Front Genet. 2022. PMID: 36110216 Free PMC article. Review.
-
Mechanisms of the FMR1 Repeat Instability: How Does the CGG Sequence Expand?Int J Mol Sci. 2022 May 12;23(10):5425. doi: 10.3390/ijms23105425. Int J Mol Sci. 2022. PMID: 35628235 Free PMC article. Review.
-
The polyG diseases: a new disease entity.Acta Neuropathol Commun. 2022 May 31;10(1):79. doi: 10.1186/s40478-022-01383-y. Acta Neuropathol Commun. 2022. PMID: 35642014 Free PMC article. Review.
References
Publication types
MeSH terms
Substances
Supplementary concepts
LinkOut - more resources
Full Text Sources
Other Literature Sources
Medical
Research Materials