Pathogenesis of Multiple Organ Injury in COVID-19 and Potential Therapeutic Strategies
- PMID: 33584343
- PMCID: PMC7876335
- DOI: 10.3389/fphys.2021.593223
Pathogenesis of Multiple Organ Injury in COVID-19 and Potential Therapeutic Strategies
Abstract
Severe acute respiratory disease coronavirus 2 (SARS-CoV-2, formerly 2019-nCoV) is a novel coronavirus that has rapidly disseminated worldwide, causing the coronavirus disease 2019 (COVID-19) pandemic. As of January 6th, 2021, there were over 86 million global confirmed cases, and the disease has claimed over 1.87 million lives (a ∼2.2% case fatality rate). SARS-CoV-2 is able to infect human cells by binding its spike (S) protein to angiotensin-conversing enzyme 2 (ACE2), which is expressed abundantly in several cell types and tissues. ACE2 has extensive biological activities as a component of the renin-angiotensin-aldosterone system (RAAS) and plays a pivotal role as counter-regulator of angiotensin II (Ang II) activity by converting the latter to Ang (1-7). Virion binding to ACE2 for host cell entry leads to internalization of both via endocytosis, as well as activation of ADAM17/TACE, resulting in downregulation of ACE2 and loss of its protective actions in the lungs and other organs. Although COVID-19 was initially described as a purely respiratory disease, it is now known that infected individuals can rapidly progress to a multiple organ dysfunction syndrome. In fact, all human structures that express ACE2 are susceptible to SARS-CoV-2 infection and/or to the downstream effects of reduced ACE2 levels, namely systemic inflammation and injury. In this review, we aim to summarize the major features of SARS-CoV-2 biology and the current understanding of COVID-19 pathogenesis, as well as its clinical repercussions in the lung, heart, kidney, bowel, liver, and brain. We also highlight potential therapeutic targets and current global efforts to identify safe and effective therapies against this life-threatening condition.
Keywords: ACE2; SARS-CoV-2; coronavirus; lung; multiple organ dysfunction; pathophysiology; therapy; viral infection.
Copyright © 2021 Lopes-Pacheco, Silva, Cruz, Battaglini, Robba, Pelosi, Morales, Caruso Neves and Rocco.
Conflict of interest statement
The authors declare that the research was conducted in the absence of any commercial or financial relationships that could be construed as a potential conflict of interest.
Figures
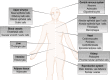
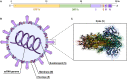
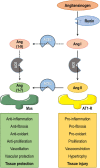
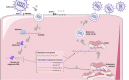
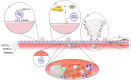
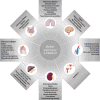
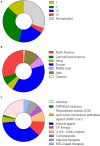
Similar articles
-
Angiotensin-converting enzyme 2 (ACE2), SARS-CoV-2 and the pathophysiology of coronavirus disease 2019 (COVID-19).J Pathol. 2020 Jul;251(3):228-248. doi: 10.1002/path.5471. Epub 2020 Jun 10. J Pathol. 2020. PMID: 32418199 Free PMC article. Review.
-
SARS-CoV-2 pandemic and research gaps: Understanding SARS-CoV-2 interaction with the ACE2 receptor and implications for therapy.Theranostics. 2020 Jun 12;10(16):7448-7464. doi: 10.7150/thno.48076. eCollection 2020. Theranostics. 2020. PMID: 32642005 Free PMC article. Review.
-
Endocrine aspects of ACE2 regulation: RAAS, steroid hormones and SARS-CoV-2.J Endocrinol. 2020 Nov;247(2):R45-R62. doi: 10.1530/JOE-20-0260. J Endocrinol. 2020. PMID: 32966970 Review.
-
COVID-19, ACE2, and the cardiovascular consequences.Am J Physiol Heart Circ Physiol. 2020 May 1;318(5):H1084-H1090. doi: 10.1152/ajpheart.00217.2020. Epub 2020 Mar 31. Am J Physiol Heart Circ Physiol. 2020. PMID: 32228252 Free PMC article.
-
Angiotensin-Converting Enzyme 2: SARS-CoV-2 Receptor and Regulator of the Renin-Angiotensin System: Celebrating the 20th Anniversary of the Discovery of ACE2.Circ Res. 2020 May 8;126(10):1456-1474. doi: 10.1161/CIRCRESAHA.120.317015. Epub 2020 Apr 8. Circ Res. 2020. PMID: 32264791 Free PMC article. Review.
Cited by
-
Subclinical Acute Kidney Injury in COVID-19: Possible Mechanisms and Future Perspectives.Int J Mol Sci. 2022 Nov 17;23(22):14193. doi: 10.3390/ijms232214193. Int J Mol Sci. 2022. PMID: 36430671 Free PMC article. Review.
-
Impacts of COVID-19 on hemostasis: coagulation abnormalities and management perspectives.Ann Med Surg (Lond). 2024 Jul 2;86(10):5844-5850. doi: 10.1097/MS9.0000000000002237. eCollection 2024 Oct. Ann Med Surg (Lond). 2024. PMID: 39359765 Free PMC article. Review.
-
A Counterintuitive Neutrophil-Mediated Pattern in COVID-19 Patients Revealed through Transcriptomics Analysis.Viruses. 2022 Dec 30;15(1):104. doi: 10.3390/v15010104. Viruses. 2022. PMID: 36680144 Free PMC article.
-
SARS-CoV-2 infection associated with monoclonal gammopathy. A case report based on the study of minimally invasive ultrasound-guided autopsy.Rev Esp Patol. 2022 Jan-Mar;55(1):41-45. doi: 10.1016/j.patol.2021.07.003. Epub 2021 Oct 13. Rev Esp Patol. 2022. PMID: 34980440 Free PMC article.
-
Kidney damage associated with COVID-19: from the acute to the chronic phase.Ren Fail. 2024 Dec;46(1):2316885. doi: 10.1080/0886022X.2024.2316885. Epub 2024 Apr 1. Ren Fail. 2024. PMID: 38561236 Free PMC article. Review.
References
-
- Arabi Y. M., Shalhoub S., Mandourah Y., Al-Hammed F., Al-Omari A., Al Qasim E., et al. (2020). Ribavirin and Interferon Therapy for Critically Ill Patients with Middle East Respiratory Syndrome: A Multicenter Observation Study. Clin. Infect. Dis. 70 1837–1844. 10.1093/cid/ciz544 - DOI - PMC - PubMed
Publication types
LinkOut - more resources
Full Text Sources
Other Literature Sources
Research Materials
Miscellaneous