A conserved PI(4,5)P2-binding domain is critical for immune regulatory function of DOCK8
- PMID: 33574036
- PMCID: PMC7893821
- DOI: 10.26508/lsa.202000873
A conserved PI(4,5)P2-binding domain is critical for immune regulatory function of DOCK8
Abstract
DOCK8 is a Cdc42-specific guanine-nucleotide exchange factor that is essential for development and functions of various subsets of leukocytes in innate and acquired immune responses. Although DOCK8 plays a critical role in spatial control of Cdc42 activity during interstitial leukocyte migration, the mechanism remains unclear. We show that the DOCK homology region (DHR)-1 domain of DOCK8 binds specifically to phosphatidylinositol 4,5-bisphosphate (PI(4,5)P2) and is required for its recruitment to the plasma membrane. Structural and biochemical analyses reveal that DOCK8 DHR-1 domain consists of a C2 domain-like core with loops creating the upper surface pocket, where three basic residues are located for stereospecific recognition of phosphoinositides. Substitution of the two basic residues, K576 and R581, with alanine abolished PI(4,5)P2 binding in vitro, ablated the ability of DOCK8 to activate Cdc42 and support leukocyte migration in three-dimensional collagen gels. Dendritic cells carrying the mutation exhibited defective interstitial migration in vivo. Thus, our study uncovers a critical role of DOCK8 in coupling PI(4,5)P2 signaling with Cdc42 activation for immune regulation.
© 2021 Sakurai et al.
Conflict of interest statement
The authors declare that they have no conflict of interest.
Figures
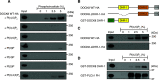
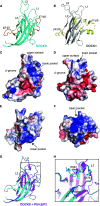
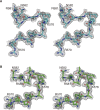
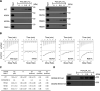
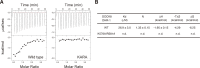
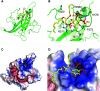
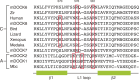
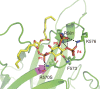
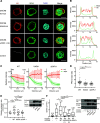
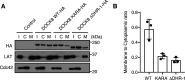
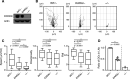
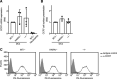
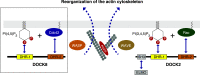
Similar articles
-
DOCK8 is a Cdc42 activator critical for interstitial dendritic cell migration during immune responses.Blood. 2012 May 10;119(19):4451-61. doi: 10.1182/blood-2012-01-407098. Epub 2012 Mar 28. Blood. 2012. PMID: 22461490 Free PMC article.
-
DOCK8 Protein Regulates Macrophage Migration through Cdc42 Protein Activation and LRAP35a Protein Interaction.J Biol Chem. 2017 Feb 10;292(6):2191-2202. doi: 10.1074/jbc.M116.736306. Epub 2016 Dec 27. J Biol Chem. 2017. PMID: 28028174 Free PMC article.
-
The pleckstrin homology domain of the Arf6-specific exchange factor EFA6 localizes to the plasma membrane by interacting with phosphatidylinositol 4,5-bisphosphate and F-actin.J Biol Chem. 2008 Jul 11;283(28):19836-44. doi: 10.1074/jbc.M800781200. Epub 2008 May 19. J Biol Chem. 2008. PMID: 18490450
-
DOCK family proteins: key players in immune surveillance mechanisms.Int Immunol. 2020 Jan 9;32(1):5-15. doi: 10.1093/intimm/dxz067. Int Immunol. 2020. PMID: 31630188 Free PMC article. Review.
-
The regulation of DOCK family proteins on T and B cells.J Leukoc Biol. 2021 Feb;109(2):383-394. doi: 10.1002/JLB.1MR0520-221RR. Epub 2020 Jun 15. J Leukoc Biol. 2021. PMID: 32542827 Review.
Cited by
-
dock8 deficiency attenuates microglia colonization in early zebrafish larvae.Cell Death Discov. 2022 Aug 17;8(1):366. doi: 10.1038/s41420-022-01155-6. Cell Death Discov. 2022. PMID: 35977943 Free PMC article.
-
CRISPR/Cas-Based Gene Editing Strategies for DOCK8 Immunodeficiency Syndrome.Front Genome Ed. 2022 Mar 17;4:793010. doi: 10.3389/fgeed.2022.793010. eCollection 2022. Front Genome Ed. 2022. PMID: 35373187 Free PMC article.
-
The inositol 5-phosphatase INPP5B regulates B cell receptor clustering and signaling.J Cell Biol. 2022 Sep 5;221(9):e202112018. doi: 10.1083/jcb.202112018. Epub 2022 Jul 25. J Cell Biol. 2022. PMID: 35878408 Free PMC article.
-
Structural biology of DOCK-family guanine nucleotide exchange factors.FEBS Lett. 2023 Mar;597(6):794-810. doi: 10.1002/1873-3468.14523. Epub 2022 Nov 4. FEBS Lett. 2023. PMID: 36271211 Free PMC article. Review.
References
-
- Adams PD, Afonine PV, Bunkoczi G, Chen VB, Davis IW, Echols N, Headd JJ, Hung LW, Kapral GJ, Grosse-Kunstleve RW, et al. (2010) PHENIX: A comprehensive Python-based system for macromolecular structure solution. Acta Crystallogr D Biol Crystallogr 66: 213–221. 10.1107/s0907444909052925 - DOI - PMC - PubMed
Publication types
MeSH terms
Substances
Associated data
- Actions
- Actions
- Actions
LinkOut - more resources
Full Text Sources
Other Literature Sources
Research Materials
Miscellaneous