Effect of Matrix-Modulating Enzymes on The Cellular Uptake of Magnetic Nanoparticles and on Magnetic Hyperthermia Treatment of Pancreatic Cancer Models In Vivo
- PMID: 33572222
- PMCID: PMC7915425
- DOI: 10.3390/nano11020438
Effect of Matrix-Modulating Enzymes on The Cellular Uptake of Magnetic Nanoparticles and on Magnetic Hyperthermia Treatment of Pancreatic Cancer Models In Vivo
Abstract
Magnetic hyperthermia can cause localized thermal eradication of several solid cancers. However, a localized and homogenous deposition of high concentrations of magnetic nanomaterials into the tumor stroma and tumor cells is mostly required. Poorly responsive cancers such as the pancreatic adenocarcinomas are hallmarked by a rigid stroma and poor perfusion to therapeutics and nanomaterials. Hence, approaches that enhance the infiltration of magnetic nanofluids into the tumor stroma convey potentials to improve thermal tumor therapy. We studied the influence of the matrix-modulating enzymes hyaluronidase and collagenase on the uptake of magnetic nanoparticles by pancreatic cancer cells and 3D spheroids thereof, and the overall impact on magnetic heating and cell death. Furthermore, we validated the effect of hyaluronidase on magnetic hyperthermia treatment of heterotopic pancreatic cancer models in mice. Treatment of cultured cells with the enzymes caused higher uptake of magnetic nanoparticles (MNP) as compared to nontreated cells. For example, hyaluronidase caused a 28% increase in iron deposits per cell. Consequently, the thermal doses (cumulative equivalent minutes at 43 °C, CEM43) increased by 15-23% as compared to heat dose achieved for cells treated with magnetic hyperthermia without using enzymes. Likewise, heat-induced cell death increased. In in vivo studies, hyaluronidase-enhanced infiltration and distribution of the nanoparticles in the tumors resulted in moderate heating levels (CEM43 of 128 min as compared to 479 min) and a slower, but persistent decrease in tumor volumes over time after treatment, as compared to comparable treatment without hyaluronidase. The results indicate that hyaluronidase, in particular, improves the infiltration of magnetic nanoparticles into pancreatic cancer models, impacts their thermal treatment and cell depletion, and hence, will contribute immensely in the fight against pancreatic and many other adenocarcinomas.
Keywords: collagenase; hyaluronic acid; hyaluronidase; magnetic hyperthermia; magnetic nanoparticles; pancreatic cancer; tumor microenvironment.
Conflict of interest statement
The authors declare no conflicts of interest.
Figures
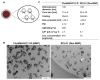
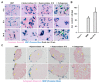
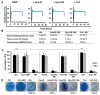
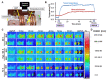
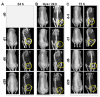
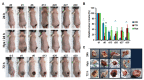
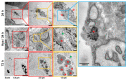
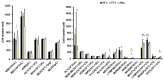
Similar articles
-
Comparison of iron oxide nanoparticle and microwave hyperthermia alone or combined with cisplatinum in murine breast tumors.Proc SPIE Int Soc Opt Eng. 2011 Feb 22;7901:10.1117/12.876535. doi: 10.1117/12.876535. Proc SPIE Int Soc Opt Eng. 2011. PMID: 24386533 Free PMC article.
-
Hadron Therapy, Magnetic Nanoparticles and Hyperthermia: A Promising Combined Tool for Pancreatic Cancer Treatment.Nanomaterials (Basel). 2020 Sep 25;10(10):1919. doi: 10.3390/nano10101919. Nanomaterials (Basel). 2020. PMID: 32993001 Free PMC article.
-
Nanoparticle-based hyperthermia distinctly impacts production of ROS, expression of Ki-67, TOP2A, and TPX2, and induction of apoptosis in pancreatic cancer.Int J Nanomedicine. 2017 Feb 7;12:1009-1018. doi: 10.2147/IJN.S108577. eCollection 2017. Int J Nanomedicine. 2017. PMID: 28223795 Free PMC article.
-
Is CEM43 still a relevant thermal dose parameter for hyperthermia treatment monitoring?Int J Hyperthermia. 2016;32(1):50-62. doi: 10.3109/02656736.2015.1114153. Epub 2016 Jan 12. Int J Hyperthermia. 2016. PMID: 26758036 Review.
-
Cancer hyperthermia using magnetic nanoparticles.Biotechnol J. 2011 Nov;6(11):1342-7. doi: 10.1002/biot.201100045. Epub 2011 Aug 26. Biotechnol J. 2011. PMID: 22069094 Review.
Cited by
-
Hyaluronan Functions in Wound Repair That Are Captured to Fuel Breast Cancer Progression.Biomolecules. 2021 Oct 20;11(11):1551. doi: 10.3390/biom11111551. Biomolecules. 2021. PMID: 34827550 Free PMC article. Review.
-
Toxicity study in a pig model of intraperitoneal collagenase as an "enzymatic scalpel" directed to break stroma in order to generate a new perspective for peritoneal carcinomatosis approach: an experimental research.World J Surg Oncol. 2022 Feb 25;20(1):53. doi: 10.1186/s12957-022-02524-2. World J Surg Oncol. 2022. PMID: 35216593 Free PMC article.
-
Iron Oxide Nanoparticles Carrying 5-Fluorouracil in Combination with Magnetic Hyperthermia Induce Thrombogenic Collagen Fibers, Cellular Stress, and Immune Responses in Heterotopic Human Colon Cancer in Mice.Pharmaceutics. 2021 Oct 6;13(10):1625. doi: 10.3390/pharmaceutics13101625. Pharmaceutics. 2021. PMID: 34683917 Free PMC article.
-
Local Magnetic Hyperthermia and Systemic Gemcitabine/Paclitaxel Chemotherapy Triggers Neo-Angiogenesis in Orthotopic Pancreatic Tumors without Involvement of Auto/Paracrine Tumor Cell VEGF Signaling and Hypoxia.Cancers (Basel). 2023 Dec 20;16(1):33. doi: 10.3390/cancers16010033. Cancers (Basel). 2023. PMID: 38201461 Free PMC article.
-
Metal-based nanomaterials and nanocomposites as promising frontier in cancer chemotherapy.MedComm (2020). 2023 Apr 4;4(2):e253. doi: 10.1002/mco2.253. eCollection 2023 Apr. MedComm (2020). 2023. PMID: 37025253 Free PMC article. Review.
References
Grants and funding
LinkOut - more resources
Full Text Sources
Other Literature Sources